For all the alien worlds that spark imaginations in sci-fi tropes, exactly how do astronomers spot planets orbiting distant stars in real life? What observational methods and equipment allow the discovery of worlds lightyears beyond our solar system?
Uncovering these exoplanets transiting stars or tugging gravitationally on their hosts reveals profound insights about alien solar systems. What advanced techniques do astronomers employ to unveil planets bound to distant stars?
In this article, you will understand how each approach for detecting worlds we cannot directly see poses its own challenges while spotting candidate planets for further investigation.
This article explores the exoplanetary hunting toolkit to answer how are exoplanets discovered across parsecs of space. Learn how spacecraft like Kepler detect faint changes in star brightness to infer orbiting planets.
Understand the rigorous verification required to confirm alien Earths and Super-Jupiters detected through these potent but indirect signatures. The search for worlds beyond the Solar System relies on some of astronomy’s most ingenious methods for unveiling hidden planetary motions.
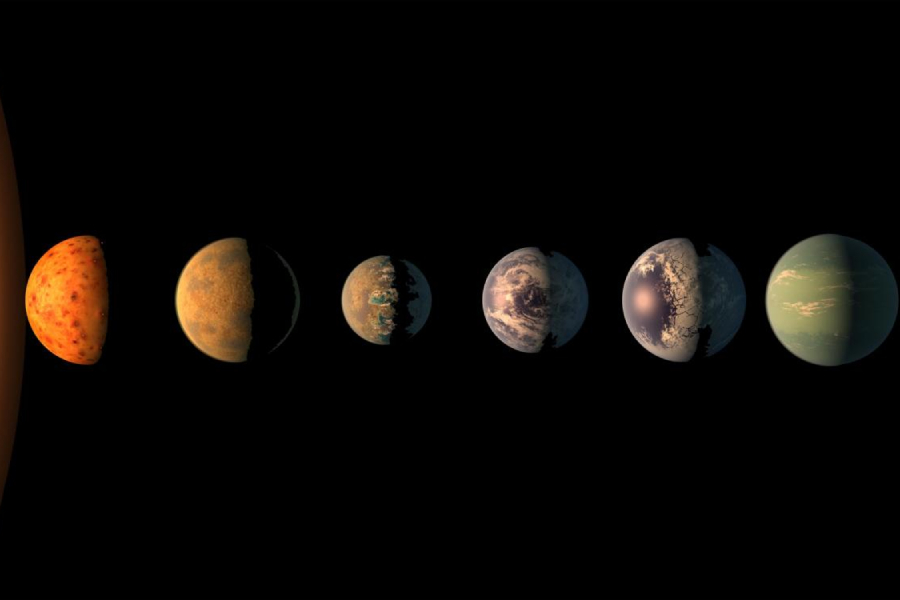
How Are Exoplanets Discovered?
How are exoplanets discovered? Exoplanets, or planets outside our solar system, are discovered using various methods. One common approach is the transit method, where astronomers observe periodic dips in a star’s brightness caused by a planet passing in front of it.
The radial velocity method detects subtle changes in a star’s spectral lines induced by the gravitational pull of an orbiting planet. Additionally, direct imaging, microlensing, and astrometry are other techniques employed to identify and study exoplanets.
Transit Method
The transit method plays a crucial role in understanding how are exoplanets detected. It relies on observing dips in a star’s brightness caused by a planet passing in front of it, blocking some of the light. These tiny dips, detected by precise instruments over regular intervals, reveal the size and orbital period of the orbiting planet.
The success story of the transit method began in the 1990s with the first definitive detections of exoplanets. Today, advanced space telescopes continue to leverage this highly successful technique. Follow-up observations help determine planetary mass by studying the gravitational effects the planet has on its host star.
Furthermore, detailed spectroscopic analysis of transits can reveal the composition of an exoplanet’s atmosphere, provided a sufficient amount of starlight passes through its outer layers during the transit.
Space missions continue to target potential exoplanets, focusing on hot Jupiter gas giants and super-Earth candidates, thanks to the valuable information provided by the transit method.
Mechanics of the transit method
Sensitive photometers precisely measure the brightness of target stars over time. When an orbiting planet transits, it blocks a small portion of starlight. Instruments record this as a periodic dip. The size and interval between dips determine the planet’s diameter and orbital period, respectively.
Multiple observations confirm that repeatable dimming comes from a transiting object. Understanding noise sources and ensuring reliable statistical analysis provides rigor to transit discovery and measurement.
Background stars and intrinsic stellar variation must not mimic an exoplanet signal. Automated software coupled with human review screens candidates before follow-up. Careful statistical validation ensures transit signals arise from planets and not imposter sources.
Determining accurate stellar parameters is crucial as well since planetary radii rely directly on precise host star radii based on models. Broadband photometry and spectroscopy provide needed measurements of stellar mass, temperature, composition, and variability.
Advantages and disadvantages
Key advantages
The transit method’s main advantages include independent planet confirmation and determining physical radius. But orbit inclination must allow edge-on viewing. Also, centered transits provide more information than grazing ones.
Host star properties can remain ambiguous without extensive supplemental observation at multiple wavelengths. Stellar limb darkening and impact parameters aside, disadvantages encompass incomplete planetary parameters absent mass.
Limitations and considerations
Transit timing variations hint at additional non-transiting bodies dynamically influencing orbits over decades. Transit spectra still require high precision to interpret atmospheric absorption signals unambiguously.
Still, the transit method yields the most exoplanets of any discovery approach, from survey missions like Kepler to TESS and ground-based detections. Multi-wavelength follow up and modeling clarifies stellar and planet densities over time after initial discovery.
By understanding the biases inherent in the transit technique for detecting exoplanets, we can broaden our understanding to include a wider variety of small worlds, even those that don’t pass in front of their host stars. So while significant drawbacks exist, the prolific productivity of transit detection provides foundations for further exoplanetary exploration.
Examples of exoplanets discovered
The Kepler Mission’s dedicated transit photometry revealed over 2,000 exoplanets.
- TRAPPIST-1 has 7 Earth-sized exoplanets that transit its ultracool dwarf star – all discovered via the transit method.
- Ground-based surveys continue the search from exoplanet factories like HAT-P-26b to oven worlds orbiting stars like Qatar-2.
- WASP-33b orbits so close to its hot host star that observations detected atmospheric metals dispersing.
- GJ 1214b orbits an M dwarf, with studies indicating a dense steamy atmosphere different from worlds like MEarth-08.
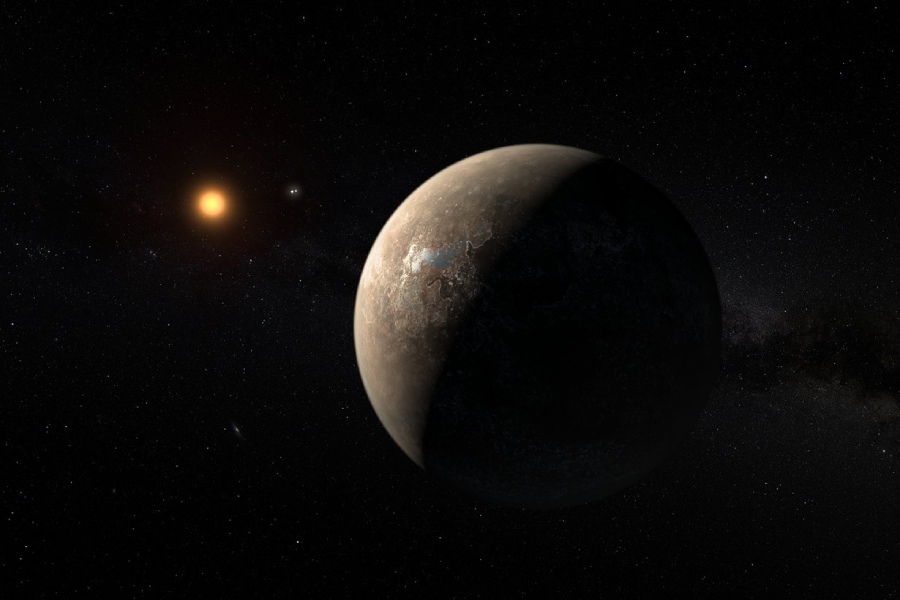
Radial Velocity Method
Transit method is only one of the methods of detecting exoplanets. Another common method to spot exoplanets is the radial velocity method. The radial velocity method detects tiny wobbles in a star’s motion caused by orbital tugs from exoplanets.
It precisely measures shifts in starlight wavelengths as the star moves toward and away from us. These minute stellar wobbles reveal planets through gravitational dance with their host star.
Astronomers apply high-resolution spectroscopy and plates. This allows them to measure stellar spectra over time. From this, they discern radial velocity shifts that indicate motion along the line of sight. Various teams focus on target stars best suited to precision Doppler measurements from the ground or space.
Technical aspects
Starlight passed through spectrographs gets measured for Doppler shifts indicating radial velocity changes. Computer analysis determines periodic stellar wobbling matching exoplanet orbits.
More massive, close-in planets create larger, faster stellar wobbles that amplify the signal. Stars must be bright and steady enough for reliable spectroscopy.
Understanding noise sources from stellar activity and instrumental errors is key. Statistical rigor ensures detected signals come from exoplanets rather than false positives. Ongoing advances in precision and stability let astronomers probe exoplanets down to Earth masses.
Advantages and disadvantages
The radial velocity method determines a planet’s orbital period and estimates its mass. But only the planet’s orbital plane inclination remains unknown. It works best for large planets orbiting close to small stars.
Activity and noise in certain stars can mask or mimic exoplanet signals. Overall the approach reliably detects many new worlds. While stellar properties can help constrain planet densities, full compositions remain ambiguous.
Without transits, images or direct spectroscopy, much planetary information is lacking. Some host stars fluctuate intrinsically or lack adequate brightness for high precision over long periods. These issues make radial velocities most productive when coupled with other techniques like transit photometry.
Examples of exoplanets discovered
- 51 Pegasi b – a hot Jupiter was the first exoplanet discovered by this method.
- Many hot Jupiters continue being found like Tau Boötis b due to their large, frequent wobbles.
- Recently Proxima Centauri c, an Earth-mass world, was spotted via precision radial velocity measurements of stellar motion.
Ground and space spectrographs anchor the discovery of gravitationally significant worlds. Barnard’s Star b is an important super-Earth discovery orbiting a nearby red dwarf. Data comes from spectrometers like HARPS.
This data provides target lists and analysis for TESS and other exoplanet missions. These missions explore the targets in more detail. They explore through complementary methods. Precise velocities unveil planets ranging from giant world Beta Pictoris c to controversial candidate Alpha Centauri Bb.
Indirect Methods
In addition to the radial velocity and transit techniques, astronomers rely on indirect observations implying the existence of exoplanets.
Gravitational microlensing utilizes Einstein’s relativity to detect planets around distant stars. It does not require observing the star or planet directly. Instead, measurements of warped background starlight unveil lensing objects like exoplanets.
Microlensing events appear rare and random from Earth’s viewpoint, as they depend on precise star alignments across parts of space. But survey missions like OGLE and MOA sample millions of stars nightly. They do this to detect significant numbers of microlensing events.
A small fraction of events are perturbed by lensing planets. Sophisticated models and analysis then determine likely exoplanet and host star properties from the light curve signatures.
The phenomenon of gravitational microlensing
Gravitational lensing occurs when foreground stars precisely align between us and more distant stars. The foreground star acts as a gravitational lens, bending and magnifying light from the background source star for months.
Planets orbiting these foreground stars can also influence the lensing, creating telltale light spikes revealing their presence statistically. Thus planetary perturbations help distinguish microlensing events from lone stars passing directly in front. Microlensing events showcase Einstein’s general relativity on galactic scales.
The technique probes exoplanet demographics in the galactic bulge and nearby galaxies like Andromeda, where distances limit other methods. Because microlensing does not rely on detecting light from the star or planet itself, it provides an independent verification channel.Â
This channel is for the existence of dark exoplanets orbiting faint stars spread throughout galaxies. This verifies exoplanets down to Mars mass. Projections expect over a thousand distant exoplanets from microlensing in the 2020s.
Conclusion
This exploration of various observational methods has shed light on how are exoplanets discovered across vast interstellar distances. By meticulously studying phenomena like gravitational microlensing, stellar radial velocity shifts and planetary transits, astronomers have confirmed over 5,000 exoplanets. Even direct imaging has helped.
Meanwhile, thousands more exoplanet candidates still await further verification. This journey of discovery is far from over. With the advent of powerful telescopes like James Webb, scientists will have the capability to analyze exoplanet atmospheres in detail, paving the way for a deeper understanding of these distant worlds.
As we continue to refine our methods and utilize advanced technology, the future holds immense promise for unraveling the mysteries of alien worlds and their potential to harbor life.