Many of us take the planets for granted, but understanding planet formation provides key insights into the origins of our cosmic neighborhood. So, how did planets form in our Solar System? Which is the oldest planet in our Solar System?
Over 4.5 billion years ago, a newborn Sun was cloaked in a primal gas and dust cloud – the raw ingredients for planets. What forces stirred this solar nebula to birth the first planetary “seeds”? Which planet formed first? Therein lies a genesis story still being uncovered – one that we will unravel to witness worlds built through cosmic collisions.
This article will overview the current scientific model of Solar System formation, describe the solar nebula, explain accretion processes, and outline open questions that research aims to solve regarding how planets formed in our Solar System.
How Did Planets Form in Our Solar System?
The leading theory is that planets formed out of a giant cloud of gas and dust around the young Sun called a solar nebula. Gravity caused this cloud’s material to clump into small bodies called planetesimals.
These planetesimals collided and combined, forming protoplanets. Further collisions enabled protoplanets to grow larger over millions of years. These protoplanets cleared their orbital paths as they interacted, merging into the four rocky inner planets and four outer gas giants.
Differences in temperature and composition across the solar nebula led to distinct types of planets. The details of this process are still being researched, like exact timescales and how planets acquired elements like water. Understanding planet formation provides key insights into the origins of our cosmic neighborhood.
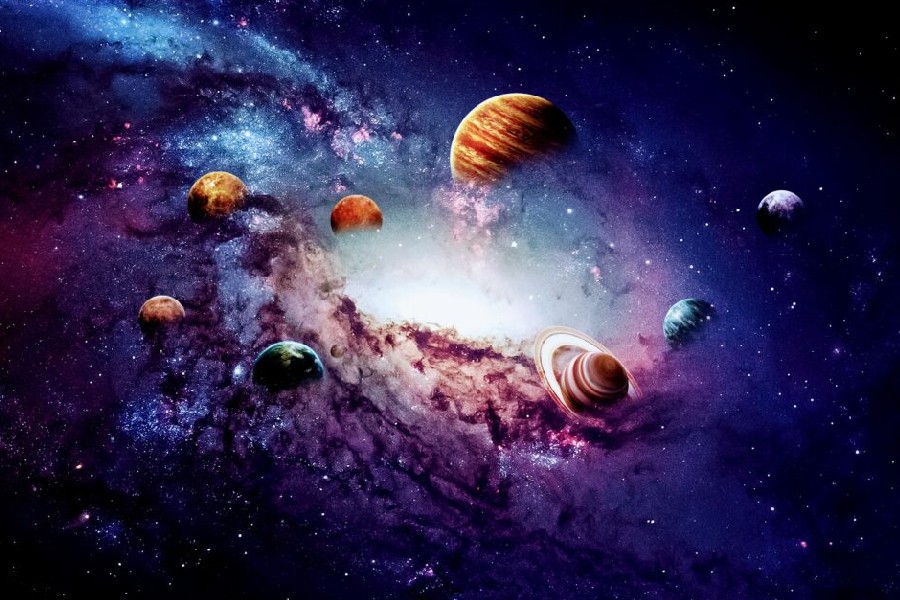
The importance of understanding planet formation
Exploring the planet formation process provides valuable insights into humanity’s cosmic origins. This knowledge not only quenches human curiosity regarding the formation of planets but also aids in discovering habitable worlds beyond our Solar System. Scientists can effectively pinpoint potential candidates that might sustain life by comprehending the conditions conducive to planet formation.
Furthermore, delving into the formation of planets in our Solar System offers valuable insights into the characteristics of exoplanets. This understanding allows for meaningful comparisons with planets orbiting distant stars. Learning about planet formation is a crucial foundation for identifying and understanding celestial bodies that may harbor life beyond our familiar cosmic neighborhood.
Definition of Planet Formation
Planet formation is the process whereby planets form out of a cloud of gas and dust that surrounds a newly formed star called a circumstellar disk. It involves celestial bodies with enough mass that the gravity is strong enough to make them spherical by overcoming the interior pressure.
These bodies form by matter aggregating within the disk until reaching hydrostatic equilibrium. It involves matter aggregating under gravity into clumps that combine into progressively larger bodies. Planet formation encompasses the stages from dust grains to fully-formed planets orbiting stars.
The Solar Nebula Hypothesis
The solar nebula hypothesis proposes that the Sun and planets formed about 4.5 billion years ago from a large spinning disk of dense gas and dust called the solar nebula. It provides a model for how the materials and conditions present within the nebula led to the beginnings of planet formation.
Understanding the solar nebula is critical to explaining the origins of planets in our Solar System. The solar nebula is thought to have originated from part of a giant molecular cloud that collapsed under its gravity.
As this segment contracted, conservation of angular momentum caused it to spin faster, flattening it into a protoplanetary disk with the protostar forming at the center. The nebula’s composition reflects the elements present in this parent cloud combined with some later contributions.
Gravity, pressure, and temperature
The gravitational field generated by the nebula’s mass and the newly ignited Sun influenced the interaction of gas and dust grains within the disk. Additionally, pressure played a crucial role, stemming from temperature variations between the hot inner nebula and the cooler outer regions.
These factors operated harmoniously, facilitating the gradual accumulation of planetesimals and protoplanets over millions of years. This process persisted until the solar wind eventually swept away the remaining gas, marking the transition to a mature Solar System.
Particles Colliding and Clumping Together
Within the nebula, small submicron particles resembling dust grains collided regularly due to interactions with the surrounding gas. These particles adhered to one another thanks to electrostatic forces, forming clumps measuring up to a centimeter.
This initial phase of particle growth marks the beginning of the process leading to planet formation in the swirling disk. As these clumps expanded, gravity played a more significant role, leading to acceleration and high-energy collisions.
The clustered particles gradually settled towards the midplane and started merging to create larger aggregate collections, reaching sizes of up to a kilometer. These sizable collections are referred to as planetesimals, representing a crucial stage in the formation of planets.
Angular Momentum and Disk Sorting
The spinning solar nebula, driven by the conservation of angular momentum, transformed into a flat disk encircling the developing Sun. Within this disk, various sorting processes were influenced by temperature variations, radiation pressure gradients, and dissimilar reactions to gas drag exhibited by light and heavy elements.
As a result, materials concentrated at specific distances, laying the foundation for the eventual amalgamation of distinct types of planets characterized by their compositions. Over millions of years, angular momentum transferred between these evolving protoplanets and the residual nebula gas, facilitating orbital migrations.
Accretion of Larger Objects in the Protoplanetary Disk
As planetesimals orbited the young Sun, collisions enabled growth into Moon-to-Mars-sized planetary embryos. With increasing gravitational pull, these embryos rapidly accumulated nearby gas and dust.
Furthermore, runaway accretion led to a few final bodies in each orbit dominating the giants Jupiter and Saturn or terrestrial planets. And Jupiter is believed to be the oldest planet in the Solar System.
The determination of final orbits over extended periods, ranging from 10 to 100 million years, is thought to be influenced by interactions such as gravitational scattering and drag within gas disks. These processes play a crucial role in shaping the ultimate paths of celestial bodies.
Additionally, the fundamental mechanism of collisional accumulation is key in the gradual growth of planets, utilizing the raw materials present in the disks that surround emerging stars. This intricate interplay of forces unfolds over significant timescales, contributing to the formation and evolution of planetary systems.
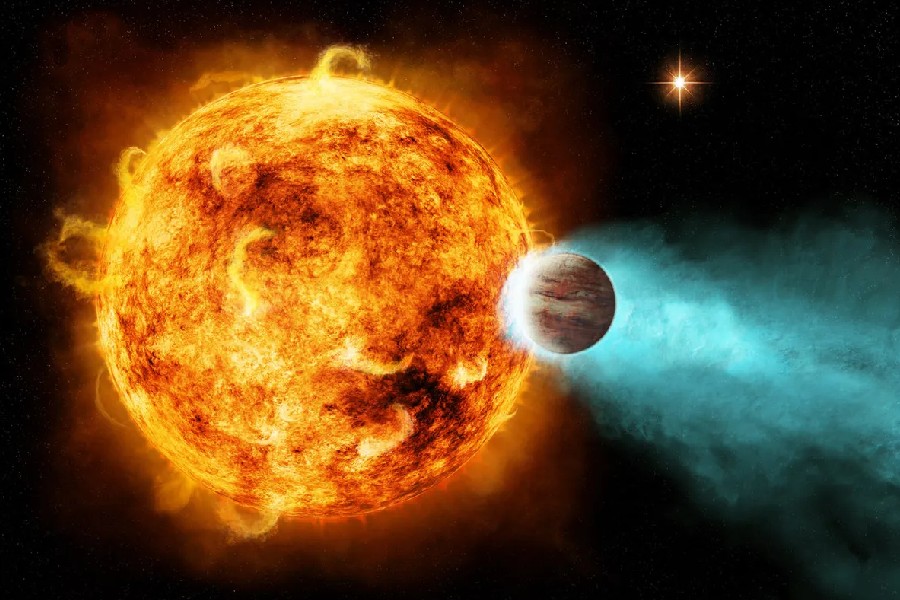
Host Stars in Planet Formation
The temperature, structure, and evolution of a protoplanetary disk, from which planets emerge, are influenced by the mass and brightness of the central star. Solar-type G stars, such as our Sun, serve as a standard reference point.
When stars are more massive, they burn hotter and brighter, leading to a quicker dispersal of disks. On the other hand, lower-mass, cooler stars result in more extended epochs for building planets.
Hence, stellar radiation and solar winds are crucial in clearing disks and halting planetary growth. It’s important to note that the composition of stars directly affects the eventual families of orbiting planets.
Other types of stars and their impact on planetary formation
Protoplanetary disks exhibit diverse properties influenced by stars, both more and less massive than our Sun. This variation affects temperature ranges and stability timeframes, leading to differences in the composition, location, and quantity of planets among various host star types.
By observing exoplanets around different stellar classes, researchers gain valuable insights that constrain models related to initial disk mass requirements. Additionally, understanding the impact of radiation and stellar winds on planet formation potential becomes clearer.
Connecting observations of protoplanetary disks to the resulting types of planets contributes significantly to the broader explanation of the factors. This enables the existence of abundant rocky worlds resembling our own Solar System.
The necessity of a host star to form planets
A host star is required to spark planet formation by providing key elements. For example, the gravitational collapse of the parent molecular cloud and the spinning up of accretion disks through the conservation of angular momentum.
Host star radiation, solar winds and supernovae shockwaves also contribute to seeding disks with heavier elements forged via nuclear fusion. Planets do not form around lone brown dwarfs or as free-floating objects.
Sustaining planetary formation
Only a true star’s substantial mass and heating can create the initial conditions and ongoing environment. Enabling seed materials to accumulate into orbiting worlds over timescales measured in millions of years.
Brown dwarfs likely lack sufficient mass, winds, and radiation. In contrast, gas cloud fragments without an anchor collapse back in on themselves or disperse.
Effects of Distant Stars on Planet Formation
In the vicinity of clustered stellar nurseries, nearby stars can disrupt forming planetary systems due to gravitational interactions. This, in turn, leads to the destabilization of planetary orbits, causing them to become more eccentric or inclined and elevating the risk of collisions. To comprehend the variety in the architecture of planetary systems, gaining insights into the dynamics of these early clusters is crucial.
When modeling flyby encounters, a delicate equilibrium emerges between stripping planets away and fostering additional collisions and mergers, ultimately contributing to the formation of larger celestial bodies. A comparison between open and globular clusters suggests that, on average, the latter may feature more elliptical planetary orbits owing to their higher density.
Violent Collision Theory for Planet Formation
Instead of gradual accretion, an alternative concept suggests rapid planet growth. This involves intense collisions between massive objects, some even ten times the size of Mars. These high-energy giant impacts might better explain the observed iron core ratios on Earth and Mars.
Supporting this idea, evidence such as the Moon’s composition resembling Earth’s mantle strengthens the hypothesis of these colossal collisions during terrestrial planet formation. Additional modeling is underway to assess the feasibility of this clashing planet scenario leading to stable orbits.
In the quest for validation, computer simulations are now focused on testing whether random crashes can accurately replicate the spacing, sizing, and circular orbits observed in the real Solar System. This approach aims to move beyond the limitations of overly excited ellipses, providing a more realistic understanding of planetary formation.
Giant Impact Hypothesis for Terrestrial Planets
The giant impact hypothesis proposes that the final stage of forming the four inner rocky worlds in the Solar System was dominated by collisions between progenitor bodies about the size of Mars. One of these impacts was a collision between a Mars-sized body and the proto-Earth.
This impact splashed off material from the proto-Earth. The splashed-off material later merged to form the Moon. The theory best explains odd Moon features like low iron content.
Achieving high enough collision velocities relies on interactions of planet-sized bodies facilitated by the presence of Jupiter. Applying this late-stage giant impact phase builds on standard models showing boulder-to-planetesimal growth earlier in Solar System evolution.
Conclusion
How did planets form in our Solar System? We have explored the leading model for how planets formed in our Solar System – starting from microscopic dust grains in the swirling solar nebula that eventually snowballed into planetesimals and protoplanets.
Through accretion and giant impacts, these protoplanets collected into the four inner rocky worlds and four outer gas giants that make up the final architecture of our cosmic neighborhood.
So, realizing the solar nebula model provides the foundation for unraveling planet formation processes across space and time. We hope this makes you appreciate the wondrous mechanisms involved in birthing worlds.