Deep within Earth, over 4,000 miles below the surface, lies a fiery, mostly iron ball over 4,000 miles wide—Earth’s core. But with no direct access to observe this deep internal structure, how do we know Earth has a core? What is Earth’s core made of? How deep is the Earth’s core?
This article delves into the intellectual journey undertaken to scientifically confirm the existence of Earth’s solid inner core. Through a meticulous exploration of various experimental, theoretical, and observational techniques, researchers unravel the mysteries veiling our planet’s core.
Navigating the intricate web of scientific endeavors is essential to comprehend Earth’s inner core temperature and delve into inner core facts. By examining these clever methods, we gain insights into how the scientific community has unveiled the Earth’s core composition and structure.
How Do We Know Earth Has a Core?
Scientists have deduced valuable insights by carefully analyzing seismic waves traversing the planet. Researchers recreated the intense pressures and temperatures in experiments on Earth’s interior.
The seismic waves from earthquakes expose density discontinuities and velocity reductions, providing crucial evidence for distinct regions within the Earth. These regions include the liquid outer core, which overlays the solid inner core and interacts with the mantle.
Moreover, scientists conducted high-pressure tests on iron-nickel alloys in experiments simulating the profound conditions of Earth’s deeper interior. These experiments matched the estimated conditions and revealed phase transitions aligning with the pressures and temperatures at the inner and outer core boundaries.
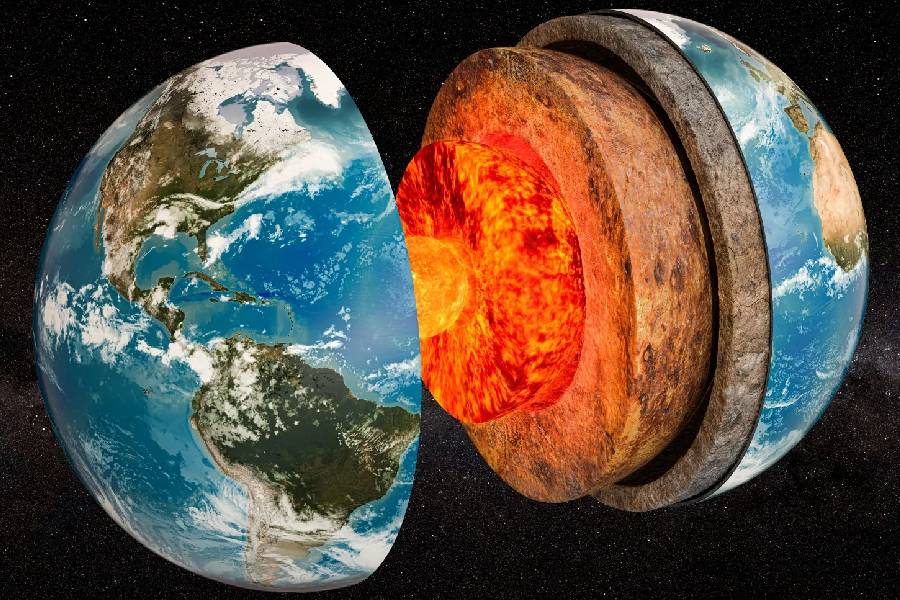
What We Know About Earth’s Core
Current understanding of Earth’s core
The planet’s core plays a crucial role in generating magnetic fields that envelop its surface. Simultaneously, it influences the movement of tectonic plates on the planet’s exterior. The core remains enigmatic and somewhat alien despite being fundamental to Earth’s processes.
Over time, significant advancements have been achieved in measuring and modeling these phenomena. However, numerous questions persist regarding the specific conditions and behaviors involved. To unravel these mysteries, additional research using diverse approaches is imperative.
Since its inception, our understanding of these planetary dynamics has expanded considerably. Nevertheless, experts continue to debate the precise origins and formation methods. Similarly, the intricate convection dynamics within the core remain a topic of ongoing scientific discussion.
Importance in planet dynamics
The core is pivotal in shaping essential Earth systems necessary for habitability. One such crucial system is the magnetic field, which acts as a protective shield against solar winds and radiation. The cycling mantle is also instrumental in bringing up elements vital for various processes.
By studying the core, researchers gain valuable insights into the planet’s earliest origins post-accretion. This exploration extends to understanding the Earth’s evolutionary trajectory over four billion years, reaching the present. Furthermore, it provides a basis for predicting future changes in interior properties.
Dynamics and complex composition emerge as critical focal points in deciphering the planet’s thermal history through proxy records and models. This approach also highlights the structural layers that develop over immense timescales. Moreover, it aids in anticipating future changes in convection currents and geological activity.
Composition and structure
Two distinct sections, the inner and outer core, have unique properties. The inner core is composed of dense iron and nickel, alloyed with lighter elemental materials that remain unknown.
The outer core has molten iron, nickel, and these light elements. However, it exhibits a more fluid state rather than a fixed crystalline lattice. Seismic observations from global sensor networks reveal these structural divisions under the crust and mantle.
Experiments probe elemental partitioning potentials between the solid and liquid phases at extreme pressures and temperatures. Complex convection and crystallization processes are also examined through computational geodynamics models.
These models are then linked back to the generation of Earth’s magnetic field over time. The models also provide insights into Earth’s past thermal evolution through gradual cooling since its formation.
Study methods
Various techniques indirectly analyze the Earth’s core, often without direct samples. Global seismic networks play a crucial role by detecting subtle density contrasts, essential for accurate modeling.Â
Additionally, experiments are conducted to simulate extreme conditions of pressure and temperature. Computational simulations are employed to model the intricate dynamics shaping the core.
Despite these efforts, direct measurement and probing remain impossible due to the immense depth. Consequently, uncertainties persist regarding the actual states and behaviors within the Earth’s core.
Interdisciplinary work
Experts yield unique insights crucial for advancing knowledge in key fields such as geophysics and geochemistry. Scientists must synthesize findings and capabilities across disciplinary boundaries to drive incremental progress on knowledge gaps.
This interdisciplinary approach is essential for effectively understanding the origins and dynamics of Earth through time. Consequently, each scientific domain contributes to the cutting edges of collective human knowledge. This collaborative effort builds a gradual understanding of the remote and extremely alien Earth core.
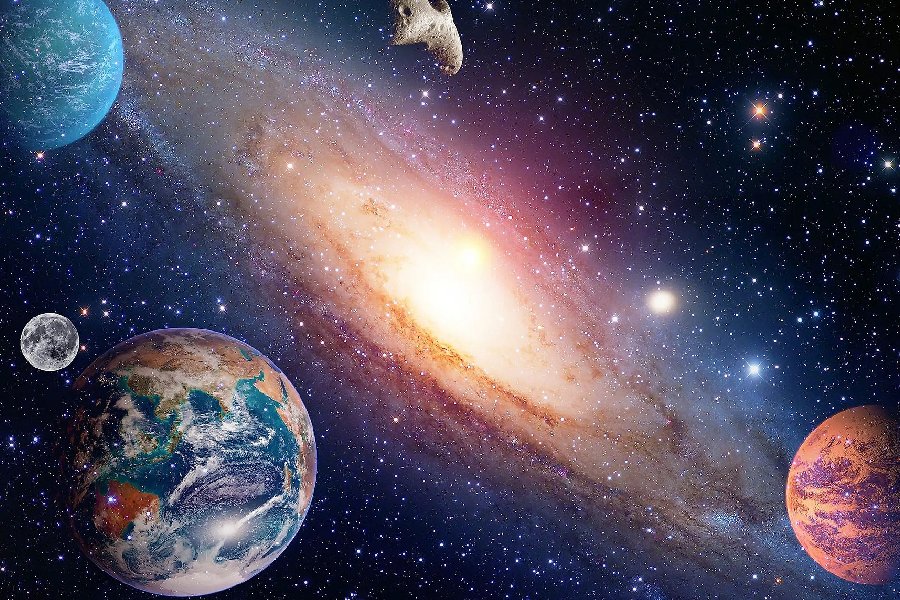
Comparing Earth to Other Planets
Earth has an outer liquid core of flowing iron and an inner solid core of crystalline iron. This unique structure generates Earth’s magnetic field, which helps protect and sustain surface life.
Planetary cores within the Solar System exhibit significant diversity in size and proportions. Also, there are hypothesized variations in the states and phases of matter believed to exist within these cores, influenced by their distinct formation conditions. Moreover, active magnetic fields’ current presence and strength vary among the planetary bodies.
Comparative insights
Mars
Mars boasts a fully molten metallic core, predominantly iron, nickel, and sulfur. Although smaller than Earth’s core in absolute terms, Mars may have hosted an ancient magnetic dynamo billions of years ago. Remnants of crustal magnetism signatures support this hypothesis.
Mercury
With its relatively large iron-rich outer core, Mercury stands out among planets due to its size. Evidence of an actively churning magnetic field in the distant past is apparent through surface magnetic anomalies. However, unlike Earth, there is no detection of current internal dynamo processes generating magnetic fields.
Jupiter
As gravitational signals indicate, Jupiter’s core is believed to surpass Earth’s entire volume. Additionally, significant convection currents are likely present within the semi-liquid hydrogen envelope. These currents fuel Jupiter’s immensely powerful and extensive magnetic field. This magnetic field serves as a protective shield for Jovian moons, some of which might contain subsurface ecosystems.
Saturn
Saturn’s core, theorized to be notably larger than Jupiter’s, surpasses Earth’s modest core size. Beneath the hydrogen mantle is a layer of metallic hydrogen, deviating from a solid inner core. Despite lacking a clearly defined solid core, Saturn maintains a robust magnetic field enveloping the planet, generated through a dynamo mechanism.
Direct Measurements of the Core
Explanation of methods used for direct measurements
Currently, no direct measurements are available for the actual core. This limitation arises from these inner regions’ extreme depths and inaccessibility beneath the surface. Instead, scientists employ indirect methods to estimate conditions.
Seismology, for instance, utilizes earthquake waves that are reflected and refracted to infer probable structural details. To examine the properties of constituent materials, sophisticated experiments are conducted, simulating expected pressures and temperatures.
In addition, complex computational models play a crucial role in understanding probable dynamics and behaviors not directly measurable by instruments. This combination of multiple tools and approaches helps build an evidence base. However, it is important to note that direct confirmation within the hostile and remote core environment is still lacking despite these efforts.
Focus on measuring seismic waves and magnetic field strength
Global seismic sensor networks, encompassing both land and sea, play a crucial role in detecting faint waves propagating through the deep planetary interior. These waves extend down to the core-mantle boundary regions, offering valuable insights into layer density contrasts. They also shed light on rigidity and viscosity changes between solid and liquid phases.
Simultaneously, worldwide magnetic field observatories meticulously monitor subtle yet meaningful changes in the Earth’s magnetic field over time and geographic space. These perturbations and fluctuations are intricately linked back to core fluid motions.
The interactions of turbulent iron alloys within immense pressures and temperatures are hypothesized to churn. This provides a deeper understanding of the complex dynamics at play.
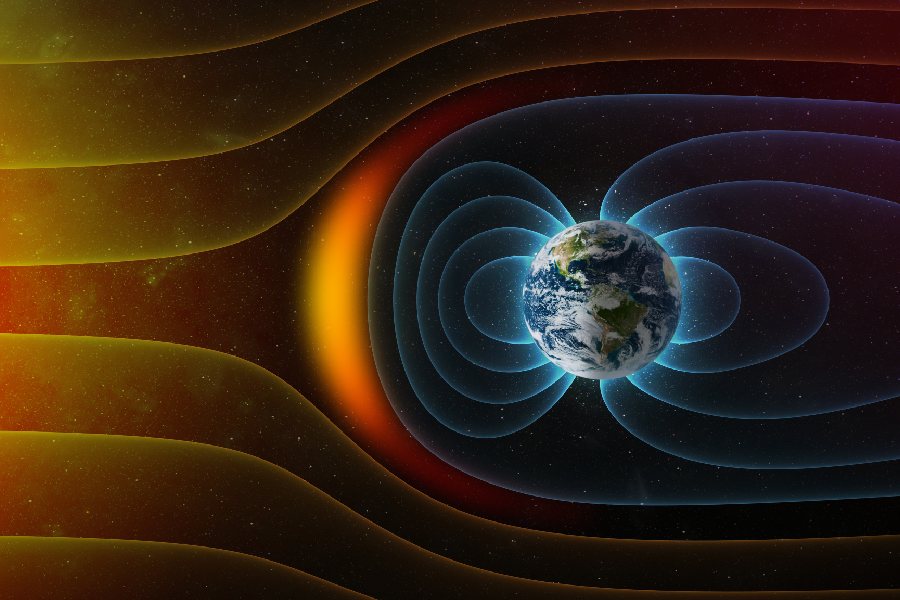
Studying the Effects of Solar Wind on Earth’s Magnetic Field
A succinct description of the effects
Cosmic rays, stemming from supernova events in distant reaches of the solar system, and charged solar wind particles, directly emitted by the Sun, consistently and perpetually approach Earth. These particles interact with Earth’s magnetosphere and global-scale magnetic field as they enter the planet.
This magnetic field plays a crucial role in deflecting and absorbing the incoming particles before they reach the atmosphere and surface. This protective mechanism shields delicate lifeforms, including humans, from the harmful effects of intense radiation.
To monitor and understand these cosmic phenomena, satellites in orbit and ground-based detectors continually measure changes in solar output over time. Additionally, they directly assess the subsequent impacts on Earth’s magnetic field’s shape, strength, and orientation.
The importance of understanding solar influences on the core’s behavior
The Sun emits varying fluxes and streams of particles, exhibiting periodic changes in intensity. As these external solar wind intensities fluctuate, they can influence Earth’s magnetic field differently.
This, in turn, leads to perturbations in the field’s geometry, which are connected to the electric currents induced in the core. Consequently, the impacts of solar wind are recognized as external triggers for observed changes in internal core processes. These internal core process variations are monitored through global sensor networks.
Analyzing Crystal Structures in Volcanic Rocks
Volcanic rocks, formed as melts rise from the Earth’s mantle, harbor tiny mineral crystals originating from the mantle and deeper regions. By examining these minuscule crystals, researchers gain valuable insights into the composition and characteristics of Earth’s core, which are otherwise challenging to sample directly.
Utilizing advanced imaging techniques, scientists can precisely identify the mineral forms of these crystals. Additionally, chemistry analysis enables the detection of elemental traces intricately captured within the crystal structures.
Through crystal analysis, the microscopic structures provide crucial clues intricately linked to the processes and conditions of core formation billions of years ago. This analysis helps narrow down competing theories regarding early core composition and models explaining the differentiation of the early Earth into distinct layers.
Insights into Earth’s core composition and characteristics
Insights from crystal analysis
The crystal structure and composition data are a valuable complement to other methods. For instance, large-scale seismic imaging of deep interiors or complex geodynamic modeling. These diverse approaches collectively contribute to building an evidence base for leading theories.
The specific mineral forms identified in the data imply very high pressures during their initial formation. The trace elements detected in the study also denote interactions with the metallic core during the initial separation from the mantle. These findings provide crucial insights into the geological processes at play.
Unraveling core mysteries
By combining data points from mineral indicators, researchers can identify potential light elements in the outer core that might impact its properties. This approach provides insights into long-term temperature variations and structural changes within the core.Â
Furthermore, it aids in unraveling persistent mysteries surrounding the inaccessible inner regions beneath the Earth’s surface. It examines the tiny yet abundant crystals transported upwards through mantle convection currents from great depths.
Conclusion
How do we know Earth has a core? By probing remote realms deep underfoot with ingenious methods, knowledge gradually advances about the planetary core—the subject of this enduring inquiry.
We highlighted the formidable challenges in remotely detecting an alien sphere more than 4,000 miles below the Earth’s surface. Various ingenious methods contribute to this complex task, including detecting echoes, signals, and proxies.
Altogether, researchers build converging lines of evidence to unravel the molten iron richness surrounding the inner crystallinity. This synthesis integrates global seismic, magnetic, and mineral observations, providing insights into early differentiation and the evolving dynamics within the Earth’s core.