X-rays are a fascinating form of light. We can’t see them with our naked eyes, yet they can reveal aspects of the universe that optical light cannot uncover. But how do X ray telescopes work? What are X ray telescopes used for?
This article will explore X ray telescope definition and X ray and gamma ray telescopes. Individuals will discover the distinctive mirrors, detectors, and focusing techniques crucial for this field.
We will also provide valuable insights into how observations in the X-ray spectrum contribute to our understanding beyond what is visible in light and other wavelengths. Continue reading to delve further into the topic to comprehend the principles underlying these potent astronomical instruments!
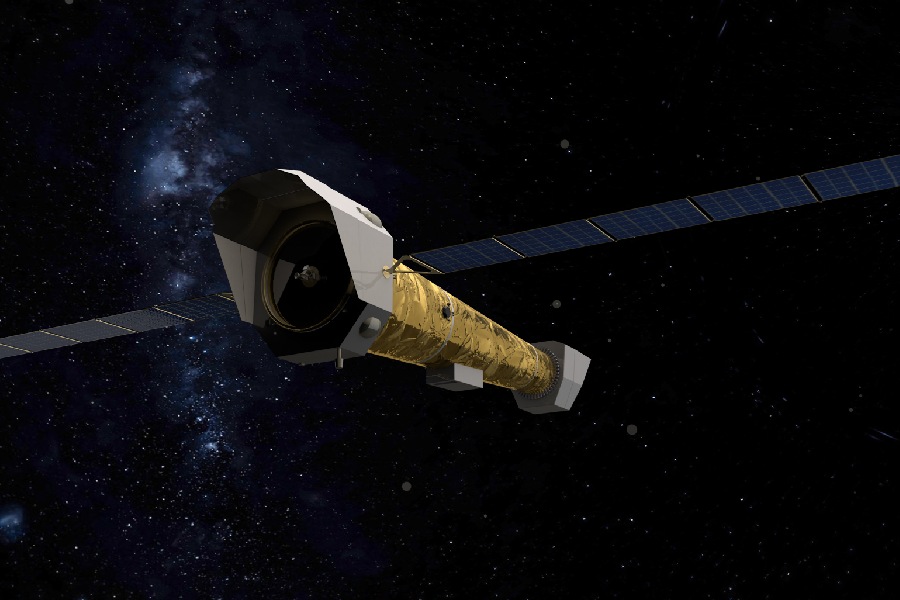
How Do X Ray Telescopes Work?
X-ray telescopes operate on detecting and imaging X-rays emitted by celestial objects in space. X-ray telescopes operate differently from optical telescopes that capture visible light.
They specifically focus on high-energy X-rays, which are invisible to the human eye. Traditional mirrors used in optical telescopes are ineffective for X-rays due to their ability to pass through them.
To overcome this, X-ray telescopes use a technique called grazing incidence. They employ mirrors with shallow angles to reflect X-rays onto detectors. These mirrors are coated with materials that enhance X-ray reflection.
The captured X-rays are then converted into electrical signals. These signals form images that reveal high-energy phenomena such as black holes, neutron stars, and active Galactic Nuclei.
How Are X-Rays Detected?
Detecting high-energy rays
Detecting high-energy rays involves specialized instruments like X-ray detectors, which capture incoming rays and convert them into electrical signals. These signals are then processed to generate insightful images, unveiling celestial phenomena like black holes and neutron stars.
Specialized detectors
X-rays, possessing significantly higher energy than visible light, necessitate specialized detectors for their capture. In X-ray telescopes, two primary detector types come into play. The first type involves gas detectors, employing inert gases.
An X-ray’s impact on gas atoms triggers electrical signals, which are then meticulously recorded for ray detection. Although gas detectors excel in precisely tracking individual X-ray photons, their drawback lies in having relatively small collecting areas.
Conversely, solid-state detectors utilize materials such as metals or semiconductors. In this mechanism, X-rays impinge upon the solid material, inducing the release of free electrons. By applying an electric field, minute currents stemming from the liberated electrons are generated and subsequently measured for ray detection.
Rapid processing
The energy of X-rays is quickly absorbed or scattered. So, the detectors and their electronics must work extremely fast. They need to record the X-ray event before its energy is lost through interactions. This allows accurate measurements of the ray properties, which is critical for analysis.
Optical vs X-Ray
Optical and X-ray detection methods fundamentally differ. Optical detection relies on visible light, employing lenses and mirrors.
In contrast, X-ray detection involves specialized detectors, such as gas and solid-state detectors, to capture high-energy X-rays. This enables distinct insights into celestial phenomena not observable through optical means.
The electromagnetic spectrum
Light comes in a range of energies called the electromagnetic spectrum. Optical light that we see makes up one small part. X-rays have much higher energies, from 100 to 100,000 times that of visible light. This makes their detection more challenging.
Interactions with matter
Visible light can be focused by lenses and reflected by mirrors. This allows sharp images. X-rays penetrate matter readily. Special grazing techniques redirect them for focusing and imaging.
Moreover, visible light triggers electrons loosely bound to atoms. But X-rays interact with tightly bound inner electrons and the nucleus.
Detection mechanisms
Optical telescopes employ CCD arrays, which accumulate images by capturing numerous photons. In contrast, X-ray detection relies on specialized sensors designed to gather individual high-energy photons.
These sensors then convert the collected photons into electric signals, facilitating subsequent processing. Distinct mechanisms accommodate the unique characteristics of high and low-energy rays.
Multi-wavelength astronomy
Optical astronomy reveals stellar properties like temperature, chemical composition, and motion. X-rays trace extreme physics like accretion disks, neutron stars, and black holes.
So, combining optical and X-ray data provides insights not accessible by either alone. Each spectrum adds pieces needed for unraveling astronomical mysteries through multi-wavelength observations.
Connecting emission mechanisms
Optical emission often arises from electron transitions in gas shells. At the same time, X-rays come from inner accretion flows, fast particle accelerations near compact objects, and extremely hot gas. Linking the different wavelength signals builds a coherent picture of the energetic processes powering cosmic emission across scale sizes.
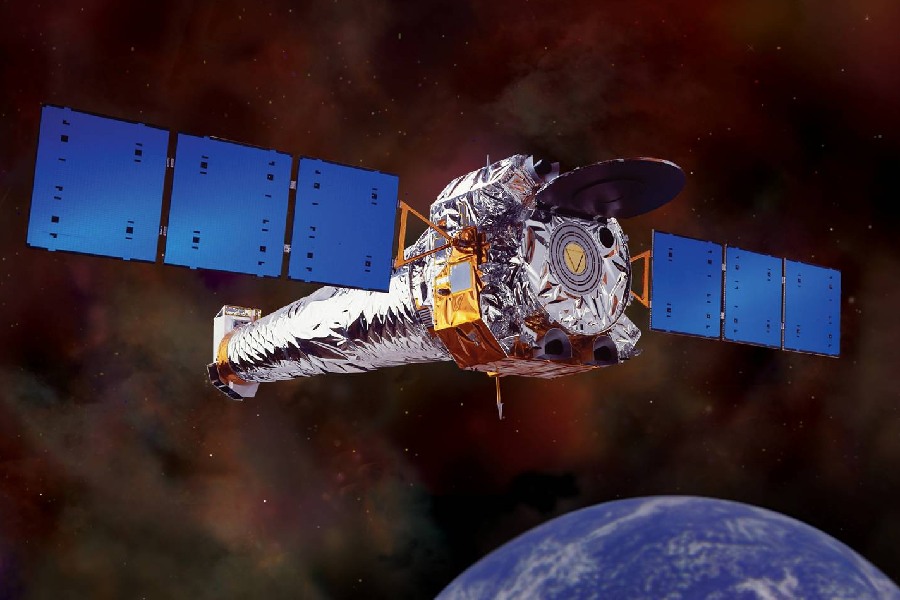
Chandra X-Ray Observatory
The Chandra Observatory is one of NASA’s great observatories. It was launched in 1999 aboard the shuttle Columbia. Chandra specializes in high-resolution X-ray astronomy and spectroscopy for studies of cosmic phenomena.
Named after astrophysicist Subrahmanyan Chandrasekhar, Chandra continues his legacy of exploring the cosmos. For example, black holes, neutron stars, dark matter and our Universe’s energetic origins.
Furthermore, Chandra carries sensitive solid-state CCD detectors. It has high-resolution mirrors made using special grazing techniques. This provides better X-ray images than previous missions. Also, the telescope points very precisely for long-duration targets.
Key discoveries and contributions
Black hole studies
Chandra observed gas swirling into black holes at high speeds. This provided evidence for the direct detection of black holes. The study also demonstrated the behavior of matter and energy under intense gravity.
Additionally, the observations helped establish limits on condensed matter states near black holes. These findings shed light on the intricate relationship between gravity and the properties of matter.
Cosmic explosions
Observing matter expelled during explosions on neutron stars, Chandra detected the creation of elements. This process involved rapidly capturing protons and neutrons within the exploded material. Furthermore, additional observations supported theories linking gamma-ray bursts to star explosions, identifying them as potential sources of gamma-ray jets.
Deep X-ray surveys
By imaging distant regions for a long time, Chandra discovered hundreds of new X-ray sources. This included new classes of black holes and neutron stars. It also detected activity from early galaxies, tracking their growth over cosmic time.
Detecting Incoming X-Rays
Advanced techniques
By employing advanced techniques, astronomers detect incoming X-rays using specialized instruments like telescopes and detectors. These tools capture and analyze X-ray emissions from celestial objects, enabling scientists to unveil crucial insights into the universe’s mysteries.
The utilization of cutting-edge methods in X-ray detection significantly propels advancements in the field of astronomy. Here are some of the important elements:
Detection elements
X-ray detectors operate by utilizing materials that generate electric signals upon impact. In their early iterations, gas cells and Geiger counters were commonly employed.
However, contemporary models have transitioned to utilizing solid-state devices such as CCDs. Despite these advancements, it is crucial to note that X-rays necessitate specialized low-noise processing electronics to effectively isolate their signals.
Detector arrays
Small individual detectors cover modest areas, but their collection area is significantly boosted when grouped into arrays. As a result, the imaging and spectroscopy capabilities improve.
The transition to multi-pixel cameras takes these advancements further, reaching megapixel scales. With large arrays, researchers can conduct wider and deeper X-ray surveys of celestial sources, enhancing the exploration of the cosmos.
Precision optics
Special near-grazing mirrors or diffractive methods are employed to enhance the precision of X-ray observations. These techniques are crucial in concentrating the X-rays onto smaller detector regions.
Therefore, a finer resolution is achieved through tighter focusing. The connection of sharp optics and large detector arrays significantly propels the field of X-ray astronomy forward.
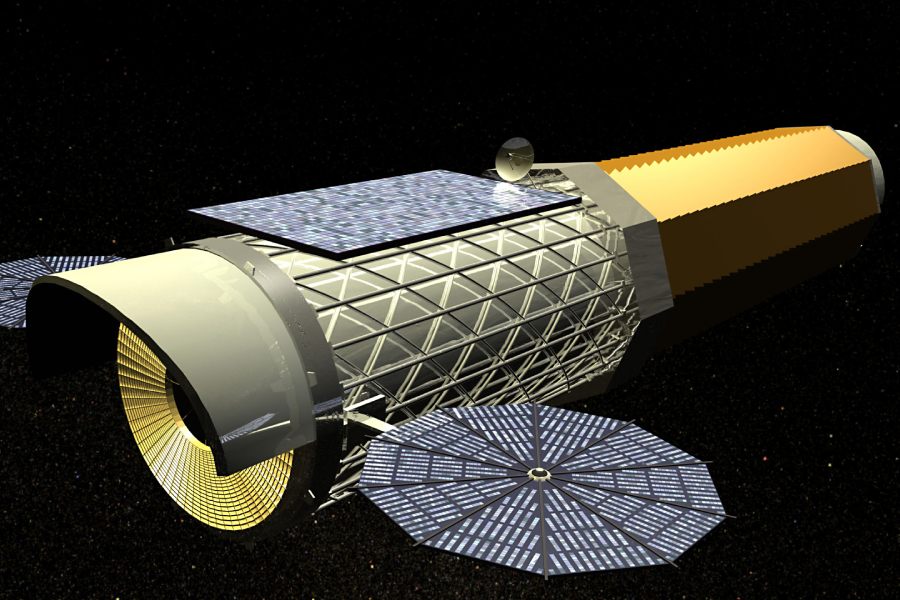
Advancements in technology that have improved X-ray detection capabilities
Electronics and processing
Enhanced X-ray measurement capabilities result from the connection of faster electronics with lower noise characteristics and increased computing power. This combination facilitates improved signal capture and the execution of complex image processing and analysis algorithms.
Consequently, integrating these advancements significantly elevates the efficiency and performance of X-ray measurements.
Improved materials
New detector materials, such as doped silicon, gallium arsenide, and cadmium telluride, have expanded their energy ranges, increased their speeds, and enlarged the areas they can effectively monitor.
In addition, these materials enhance the response to soft X-rays. Moreover, applying new thin film coatings on optics contributes to improved reflectivity. So, these advancements directly result in gains in sensitivity.
Grazing incidence methods
Adjustable cylinder substrates in specialized mirror fabrication enhance grazing angles, improving finger X-ray focusing. Hence, imagery and spectrometry attain sharper precision. The reflectivities have reached 50 to 80% for low X-ray energies, with ongoing advancements anticipated for high-energy X-rays.
How Are Data From Incoming Rays Analyzed?
Unraveling the cosmos
Unraveling the cosmos involves meticulously analyzing data obtained from incoming X-rays, a pivotal step in scientific discoveries. This process unveils crucial insights into celestial phenomena, enabling researchers to comprehend the intricacies of the cosmos and broaden our understanding of the universe’s vast mysteries.
Processing pipeline
Initially, the raw detector data undergoes a crucial filtering and cleaning process. This step is essential for removing defects and eliminating any unwanted noise artifacts. Subsequently, specialized software is employed to reconstruct events, transforming them into coherent images and spectra.
Additionally, there is an option to integrate multi-wavelength data, enhancing the comprehensiveness of the analysis. Lastly, scientists meticulously interpret the refined data, utilizing established models to derive meaningful insights.
Signature analysis
Various elements and physical processes imprint distinctive “signatures” on X-ray signals. Researchers can pinpoint the emission sources by scrutinizing spectral lines, variability, and band intensity. Also, comparing these observations with predictions facilitates the identification of specific cosmic origins.
Pattern recognition
The utilization of image analysis software is crucial in identifying various astronomical phenomena. This software can recognize extended structures such as gas clouds and relic radiation patterns.
Furthermore, it excels in pinpointing compact regions that may indicate the presence of black holes or neutron stars. By automating the feature recognition process, the pace of discovery is significantly accelerated. This innovative approach enhances efficiency and allows researchers to uncover celestial objects and patterns faster and more precisely.
Notable breakthroughs or insights gained through data analysis
Black hole spin
In the exploration of black holes, detailed spectroscopy has unveiled fascinating findings. By closely examining Doppler-shifted iron lines, scientists have gained crucial insights.
Moreover, analyzing the broadening and shapes of these spectral lines has offered compelling evidence supporting the idea that black holes spin at remarkable speeds. This discovery not only enhances our understanding of these enigmatic cosmic entities but also validates essential predictions derived from the principles of general relativity.
Dark matter mapping
By subtracting expected gas signatures from spectral data, residual maps emerged, indicating dark matter. This invisible matter dominates galaxies and clusters, bending light through lensing effects seen in X-ray images.
Star life cycles
X-ray spectroscopy is able to determine compositions, temperatures, densities and velocities of stellar remnants and gas clouds. This helps establish evolutionary timelines as stars progress through supernovas, neutron stars, black holes and beyond.
Conclusion
How do X ray telescopes work? As explored, X-ray telescopes employ ingenious specialized optics, detectors, and data processing tailored specifically to observe the Universe in high-energy X-ray light invisible to human eyes.
By unraveling the encoded signatures in emissions from exotic environments, these observatories grant vision into intense phenomena around black holes, supernovae, and more. The complex inner workings make this powerful vision inaccessible to conventional telescopes.
So, engineers venture into uncharted territories and constantly encounter deeper mysteries waiting to be unraveled. They achieve this by skillfully manipulating glass lenses and the entire electromagnetic spectrum.