Telescopes demonstrate impressive abilities to make distant celestial objects observable with your eye. But how do they do that? What are the parts of a telescope, and how do they capture photos from distant stars and direct light into your curious eye?
We’ll explain the equipment and components, from basic backyard stargazing telescopes all the way up to giant observatory telescope arrays, that make astronomy possible. The same core optics empower both simple backyard and complex research instruments.
Discover how each critical telescope piece holds a vital role in collecting, accurately focusing, and channeling light to reveal profound cosmic secrets from distant stars. Join us as we explore what gives an optical telescope its functionality, from the structural supports to the precisely aligned mirror.
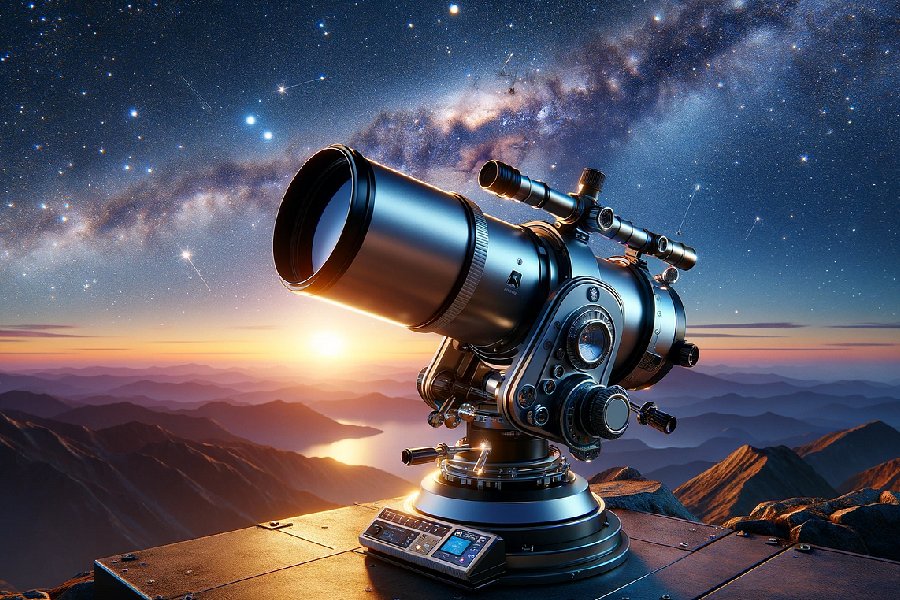
What Are the Parts of a Telescope?
So, what are the parts of a telescope and their function? A telescope comprises key and additional components for celestial observations. The main telescope components are: light-gathering objective lens or mirror, eyepiece, focuser, and protective tube.
Additional elements, such as the finder scope and diagonal, assist in object location and comfortable viewing. The mount supports controlled movement, with options like equatorial and alt-azimuth mount.
Together, these components form an integrated system housed on a tripod or mounting base to explore the wonders of the universe efficiently.
The primary mirror
Light collection properties
The primary mirror is the largest component in reflecting telescopes. It collects and focuses incoming light toward a focal point. Size correlates to light-gathering power.
Large objective mirrors are key to maximizing a telescope’s light-gathering ability. Precision grinding and optical polishing are used to properly shape these large mirrors, enabling them to capture more of the dim celestial light coming from distant stars.
Bigger primary mirrors can exponentially improve a telescope’s ability to observe faint, faraway stars and galaxies. This makes large mirrors crucial for advanced telescopes used in astronomy and cosmology research of the deep cosmos beyond our stellar neighborhood.
Purpose and variations in design
Parabolic-shaped reflector primaries concentrate diffuse light from celestial sources. Solid glass blanks get finely polished and engraved to precision contours. Alternate materials like metals or liquid mercury allow large, thin, lightweight mirrors.
Mirror design balances factors like weight, rigidity, and thermal stability to avoid distortion upon slight temperature shifts, which would degrade images. New advancements in spin casting and mirror support cell technology are helping improve the fabrication of telescope components.
The secondary mirror
Redirecting light
The secondary mirror is smaller and redirects the light concentrated by the large primary mirror. This focused light is sent into the eyepiece for magnification.Â
The secondary mirror must be precisely aligned at a 90-degree angle to the primary mirror’s optical axis for the sharpest image. This layout, with a smaller secondary mirror reflecting the eyepiece, allows for building more compact, portable Newtonian telescopes.
The two mirrors form a properly oriented image for the observer rather than an inverted reversal. The smaller secondary mirror blocks some light but allows for smaller, portable telescopes versus fixed observatory ones. This enables transportable stargazing by trading off light-gathering power.
Advanced configurations
Other telescope designs like the Cassegrain or Gregorian use a curved, convex secondary mirror. This allows the light to be folded into a zigzag path rather than a straight line. The folded path enables very long focal lengths to fit into compact tubes rather than extremely long telescopes.
Creative curvature combinations allow large primary mirrors to be packed into smaller overall packages compared to simpler reflector layouts. Simple reflectors need long empty spaces between the main mirror and where the image is formed.
More mirrors also means more light gets absorbed rather than reflected to the eyepiece. So extra precision and careful mirror polishing helps maximize the light delivered for photography and instruments relying on ample light for analysis tasks.
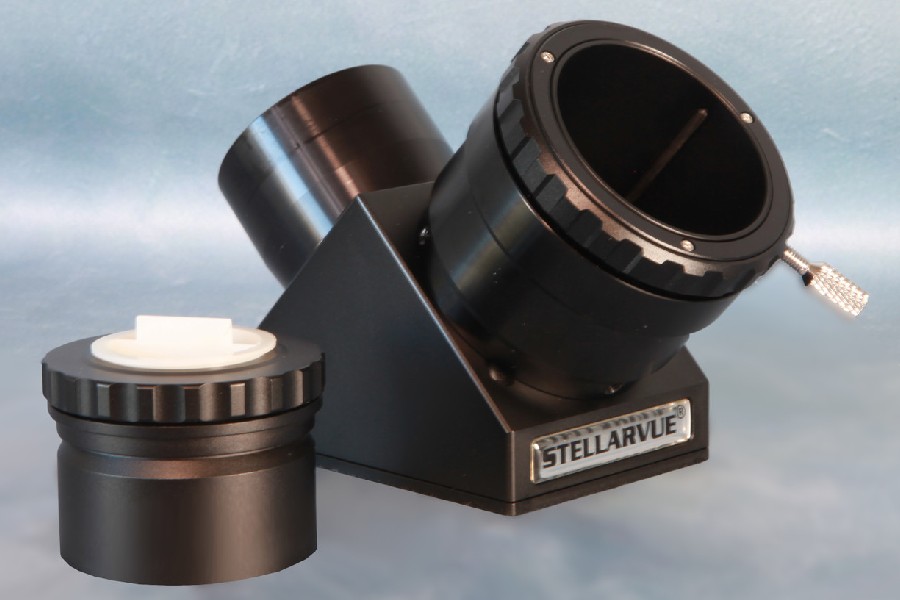
Star diagonal mirrors
Understanding the use of star diagonal mirrors
Star diagonal mirrors bend incoming light at an additional reflection, usually angling 90 degrees relative to the main optical path. This enables more comfortable ground-based eyepiece aiming while tracking celestial objects across the sky.
Tilting light paths lets observers keep their backs straight while analyzing the skies instead of craning their necks upward. This ergonomic flexibility allows longer comfortable periods at the eyepiece without strain or soreness.
Role in changing the direction of the view
Right-angle star diagonal mirrors deviate the tilt of observed sights accommodating horizontal telescope tube assemblies. Redirecting facilitates convenient, neck-pressure-free tracking versus constantly peering straight up through non-deviating designs.
Adjustable diagonal mirrors also suit optimizing illumination across varied eyepieces that may function best at specific orientations to incoming beams. Adapting angles prevents vignetting or uneven exposure.
Objective lenses
Objective lenses serve as entry portals converging observed light in refracting telescopes before traveling to meet awaiting eyepieces. Performance relies on precision optics to gather and concentrate diffuse illumination with minimal aberrations.
Well-figured lens curvature minimizes optical distortions, so stars focus on tight points instead of blurred blobs. Quality objectives remain essential components determining ultimate resolving capabilities.
Types of objective lenses
Common elementary objective lens types include simple convex, concave, and meniscus telescope designs. More exotic solutions minimize chromatic and spherical aberrations via apochromatic combinations with higher refraction index crown glass coupled with lower index flint glass.
Upgraded objectives better concentrate wavelengths into the same focal point. Expensive fluorite or apochromatic-refractor telescopes deliver pinpoint star images for astronomical photography.
Glass lens components
Lens role
Glass lenses diffuse light from the heavens using transparent substrates carefully shaped by grinding/polishing. They then get precisely aligned within optical assemblies along the observation path, culminating at eyepieces.
Proper lens spacing as photons pass creates constructive interference, yielding magnified, focused, and crisp images revealing structure and details about astronomical objects of interest.
Design priorities balance light-bending power against internal flaws causing visual aberrations degrading image output sent to detectors.
Variations in glass lenses
Standard crown silicate provides basic glass but varies in exact recipe balancing factors like clarity, durability, and temperature response. Adding lead boosts light bending power.
Rare earth elements also raise the refractive index rating, further minimizing chromatic aberration in premium optics suited for research, discovery, and photography objectives.
Specialized low-expansion substrates like Pyrex and spin-cast ceramics achieve exceptional thermal stability for precision focusing during temperature changes over long-exposure photography sessions.
Types of glass lenses
Common glass lens types balance clarity, hardness, stability, and cost factors. Soda-lime silicate crowns offer baseline quality, while barium crowns cost more but perform better. Flint glass mixes improve light bending, augmenting achromatic performance at added expense. Exotic glass ceramics boast enhanced thermal properties over traditional glasses.
Curved mirrors
Contour shapes
Shaped mirror curvature focuses arriving light to a singular point. Concave spherical bowing works well but induces some aberration.
Alternative parabolic front contours better concentrate rays minimizing distortion especially in wide-field reflector telescopes. This shape eliminates optical errors found in spherical models.
Applications of curved mirrors
Primary reflector mirrors catch and redirect diffuse illumination towards awaiting secondary mirrors. Together the combination produces focused exiting beams ideal for magnification by eyepieces or captured imagery via instruments like astronomical CCD cameras.
Smaller secondary mirrors also rely on calculated curvature to accurately re-reflect concentrated light from the main primary reflector dish to the exit focal point and awaiting detector instrumentation.
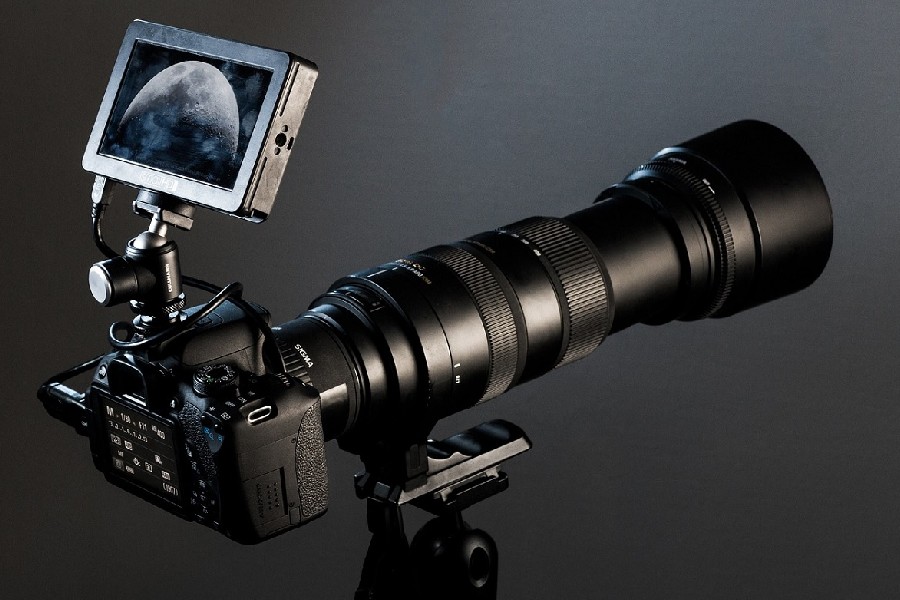
Conclusion
As we conclude our exploration of what the different parts of a telescope enable, we hope studying primary mirrors, specialized lenses, essential optics, and supporting components has enlightened your perspective on the instrumentation enabling cosmic observation.
This article aimed to provide fundamental knowledge so you understand telescopes better as scientific tools revealing our universe’s marvels and mysteries.
Whether reflecting incoming light or refracting it to catalyze new discoveries, now grasp internally how telescope concentrates. It concentrates through objectives, eyepieces and telescopic configuration to deliver revelations unveiled across space and time.
Just like how Galileo changed our understanding of light from the stars over 400 years ago, may your newfound knowledge of telescope parts inspire you to passionately explore and sicvoer astronomical insights for many years to come, illuminated by the wonders of the celestial world.