Among the most dramatic events in our universe, supernovae signify the explosive deaths of stars. These luminous stellar explosions outshine entire galaxies and lead to the formation of neutron stars or black holes. But what causes supernova?
In this article, we will explore the critical factors and physical processes behind supernovae. We will compare and contrast the specific mechanisms of Type Ia versus Type II supernovae categorized based on spectral patterns.
Along the way, we will visualize interior views of massive stars transitioning into remnants like pulsars and magnetars. Let’s unravel what ultimately causes stars to end their stable lives in cataclysmic supernovae.
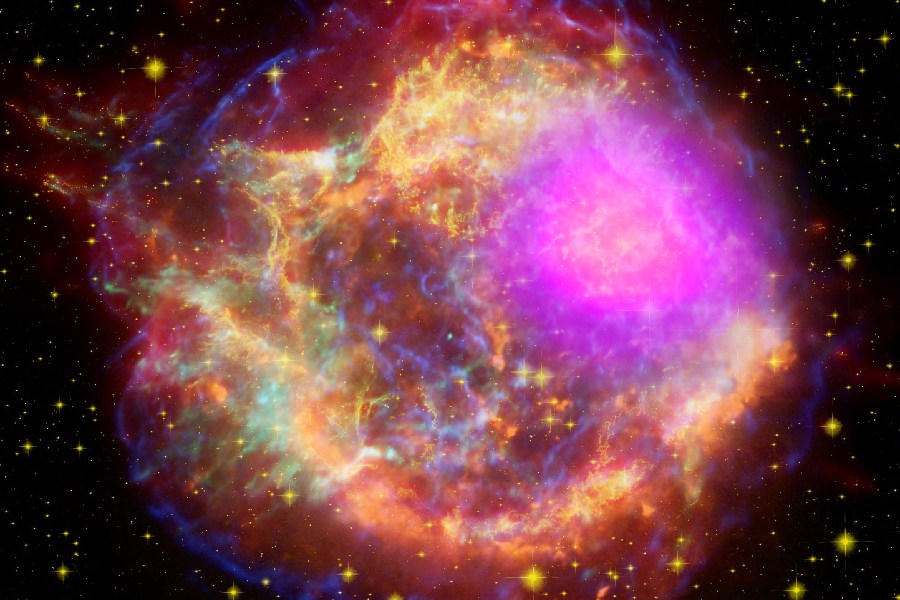
What Causes Supernova?
Supernovae result from two main mechanisms: the death of massive stars or the explosion of a white dwarf in a binary system. In Type II supernovae, the core of a massive star collapses under its own gravity when it runs out of nuclear fuel, triggering a powerful explosive release of energy.
On the other hand, Type Ia supernovae occur when a white dwarf in a binary system accretes matter from its companion star until it reaches a critical mass limit. This leads to a runaway thermonuclear explosion.
Stellar Evolution and End Stages
The life cycle of stars
Stars form when clouds of gas and dust collapse due to gravity. As stars grow older, they convert lighter elements into heavier ones through fusion for energy. Smaller stars eventually fade away as white dwarfs, while larger ones end dramatically with supernova explosions.
The occurrence of a supernova hinges on factors like the star’s mass and its developmental journey. Nevertheless, all supernovae entail a massive restructuring of the star’s core, culminating in a spectacular explosion that scatters heavy elements throughout space.
But why do stars go supernova? What causes supernova? We will dive deeper into this matter.
Scenarios leading to supernova explosions
There are two main types of supernova explosions – thermonuclear and core collapse. Thermonuclear supernovae happen when white dwarfs accrue matter from a companion star until they reach a critical mass limit and detonate.
On the other hand, core collapse supernovae signal the end of very massive stars. As the stellar core runs out of nuclear fuel, it can no longer resist the weight of the outer layers. So, the core implodes, triggering an explosion of the star’s outer shells.
Core Collapse Supernovae (Type II)
Core collapse process
Massive stars, those more than eight times the mass of our Sun, generate heavier elements via nuclear fusion in their cores. Initially, they fuse hydrogen, progressing to helium, carbon, oxygen, neon, silicon, and iron.
As the star exhausts its fuel, its core contracts and heats up, initiating the fusion of the succeeding heavier element. This cycle persists until the core predominantly consists of iron, unable to generate energy through fusion.
The iron core is sustained by the pressure exerted by densely packed electrons. As the core accumulates mass from its surroundings, it reaches a pivotal threshold known as the Chandrasekhar limit, approximately 1.4 times the Sun’s mass. Upon reaching this limit, the electron pressure can no longer counteract the core’s gravitational force, leading to its collapse.
Overview of mechanisms leading to core collapse
Several factors contribute to the collapse of the iron core in massive stars. One key factor is the loss of energy through neutrinos.
As the core gets denser and hotter, high-energy light particles interact with atomic nuclei and electrons, creating neutrinos. These neutrinos escape from the core, taking energy with them and gradually cooling the core, reducing its pressure.
Another factor is electron capture. In the extremely dense core, electrons are forced into protons, creating neutrons and releasing neutrinos.
This reduces the number of electrons that can push against the core’s collapse. The combination of neutrino cooling and electron capture eventually causes the iron core to collapse catastrophically under its gravity.
Triggers for core collapse
Several critical factors influence the onset of core collapse in massive stars. One trigger is the exhaustion of nuclear fuel in the core. When the star has fused all its core material into iron, no further energy can be generated through fusion.
This loss of energy production means that the core can no longer support itself against the inward pull of gravity. Another factor is the increasing mass of the iron core. As the star continues to fuse elements in the shells surrounding the core, the core grows massively.
When it approaches the Chandrasekhar limit of about 1.4 solar masses, electron degeneracy pressure becomes insufficient to counteract gravity. The core then begins to collapse under its weight.
Overview of the processes leading to Type II supernovae
The collapse of the iron core marks the beginning of a Type II supernova. As the core collapses, it reaches incredible densities and temperatures.
The collapse continues until the core reaches nuclear densities. At this point, the strong nuclear force provides a sudden and intense resistance to further compression. This abrupt halt to the collapse generates a shock wave that propagates outward through the star’s outer layers.
The shock wave initially stalls due to nuclear dissociation and neutrino emission energy losses. However, a small fraction of the neutrinos produced during the collapse interacts with the stellar material behind the shock front.
This neutrino heating reinvigorates the shock wave, allowing it to continue its outward propagation. The shock wave eventually reaches the star’s surface, ejecting the outer layers in a spectacular explosion known as a Type II supernova.
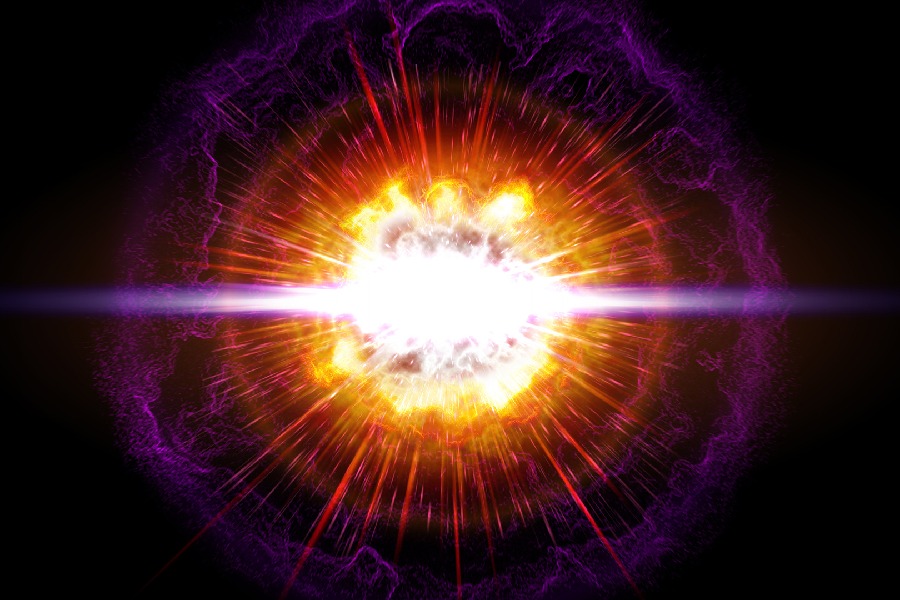
Type Ia Supernovae
Unique characteristics
Type Ia supernovae have some distinctive features that set them apart from other stellar explosions. They have consistent peak luminosities and uniform light curves.
These qualities make Type Ia supernovae very useful for making distance measurements in astronomy. Observations of Type Ia supernovae at varying distances have led to major discoveries about cosmic acceleration and dark energy.
Progenitor systems
It is believed that Type Ia supernovae originate from binary star systems containing a white dwarf. The white dwarf accretes material from its companion star until it exceeds the Chandrasekhar limit of about 1.4 solar masses. The properties of the companion star and the accretion process play an important role in laying the foundations for a Type Ia supernova.
Ignition process and explosion mechanisms
As a white dwarf collects mass from a companion star, its core becomes denser, leading to higher temperatures. Eventually, this can trigger carbon fusion at the core, often under degraded conditions.
Then, rapid thermonuclear reactions occur, turning material into radioactive nickel and iron peak elements, causing the star to explode. There is ongoing debate about whether the initial ignition happens as a subsonic deflagration before shifting into a supersonic detonation during most of the burning process.
Nuclear Fusion and Element Synthesis
Nuclear fusion reactions
Nuclear fusion is the process that drives energy production in the cores of stars. It happens when lighter elements combine to form heavier ones, releasing energy.
For example, hydrogen combines to form helium, further fusing into carbon, neon, oxygen, and silicon as the star progresses through its life stages. These fusion reactions within a star’s lifespan are crucial for creating elements ranging from hydrogen to iron.
Role of supernovae in element dispersal
Supernova explosions scatter heavy elements formed during the later stages of a star’s life or through explosive reactions. In Type Ia supernovae, radioactive nickel-56 transforms into iron-56 as it decays.
Core collapse supernovae create even heavier elements in their powerful shock waves by bombarding nearby material with neutrons. These explosions distribute these elements into space, enriching the interstellar medium for the formation of new stars and planets in the future.
Supernova Remnants and Aftermath
Supernova remnants
Supernova remnants are the expanding clouds of gas and dust that remain after a supernova explosion. These remnants are characterized by their complex structure and high-energy radiation.
They consist of the ejected material from the progenitor star, as well as the surrounding interstellar medium swept up by the powerful blast wave. Supernova remnants are typically observed in various wavelengths, including radio, optical, and X-ray.
They exhibit distinct features such as bright filaments, shock fronts, and hot, ionized gas. These features provide valuable insights into the dynamics and energetics of the supernova explosion.
Role in galactic ecosystems
Supernova remnants play a crucial role in shaping the galactic ecosystem. They act as cosmic recycling centers, dispersing heavy elements forged in the progenitor star’s core back into the interstellar medium. This enrichment process is essential for the formation of new generations of stars and planets.
Moreover, supernova remnants are responsible for accelerating cosmic rays, which are high-energy particles that permeate the galaxy. The shock waves generated by the expanding remnants can accelerate particles to tremendous energies, contributing to the overall cosmic ray flux.
Aftermath of supernova explosions
After a supernova explosion, what happens to the remaining stellar core depends on how much mass it had at the start. If the star had a mass between eight and 20 times that of our Sun, the core might shrink to form a neutron star. Neutron stars are very dense and made up entirely of neutrons.
However, if the original star was heavier, over 20 times the mass of our Sun, its core’s gravity could be too strong. This could cause the core to collapse into a black hole. Black holes are areas in space where gravity is incredibly powerful, pulling in everything, even light, and not letting it escape.
Contributions of supernovae to the enrichment of the interstellar medium
Supernovae play a vital role in enriching the interstellar medium with heavy elements. Through nuclear fusion processes, stars fuse lighter elements into heavier ones during the stellar life cycle.
When a massive star undergoes a supernova explosion, these newly synthesized heavy elements are expelled into the surrounding interstellar medium. This enrichment process is crucial for the formation of new stars and planetary systems. The heavy elements ejected by supernovae provide the raw materials for forming rocky planets.
Like Earth, these rocky planets are composed of heavier elements. The enrichment from supernovae also contributes to the diversity of chemical compositions observed in the universe.
Conclusion
What causes supernova? We hope this article helped you understand better the awe-inspiring phenomenon of supernovae and their profound impacts on the cosmos. These cataclysmic stellar explosions mark the dramatic ends of massive stars’ lives, leaving behind spectacular remnants that shape galactic ecosystems.
From seeding the interstellar medium with heavy elements to forming exotic objects like neutron stars and black holes, supernovae are fundamental drivers of cosmic evolution. Unraveling the mysteries behind these celestial events not only deepens our knowledge of the universe but also sheds light on our origins, as the elements forged in supernovae are the building blocks of life itself.