Stars stand out in the night sky with their unique glimmering shine. These countless fiery orbs have brightened the night for eons. But when gazing at their majestic splendor, have you ever wondered about their composition? What is a star made of? What makes the star shine so bright? What raw material came together from across the cosmos to create these radiant celestial spheres?
As we crack open the mysteries within colorful stellar nurseries and blazing stellar cores, an intriguing answer emerges on what distant suns and our own life-giving Sun are made of. You’ll discover the unique fusion ingredients found at the blazing-hot cores of stars and learn how scientists study distant fiery compositions.
Understanding stars’ inner workings tells intriguing scientific stories about our universe’s origins. The insights ahead showcase how the elements in stars and human life ultimately have a shared cosmic history.
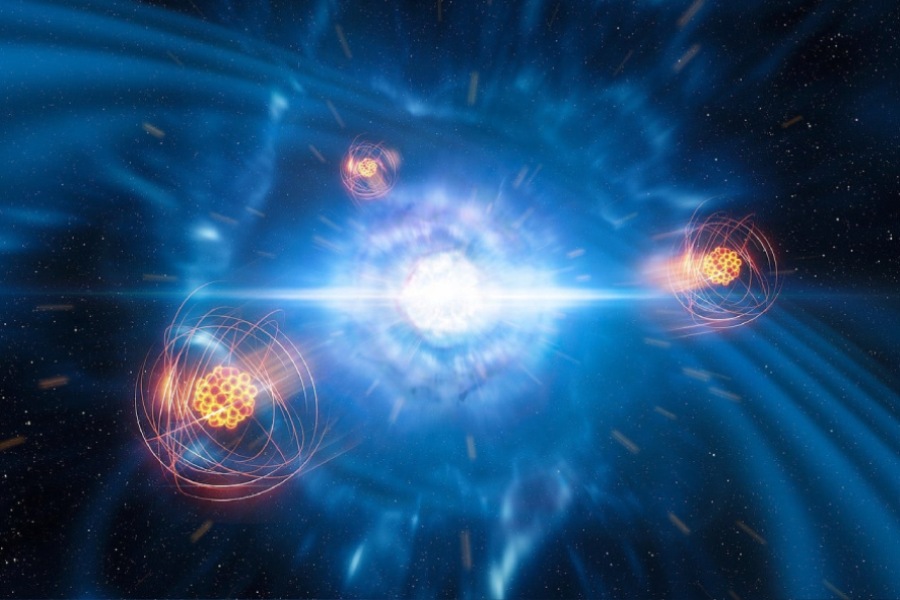
What Is a Star Made Of?
Stars are primarily composed of hydrogen and helium, the two lightest elements in the periodic table. The immense gravitational pressure at the core of a star triggers nuclear fusion reactions. In these reactions, hydrogen atoms fuse together to form helium.
This fusion process releases tremendous amounts of energy. This energy, in the form of light and heat, sustains the star’s luminosity and prevents it from collapsing under its own gravity.
Additionally, stars may contain trace amounts of heavier elements, which are produced through nuclear fusion during the star’s life cycle or released into space during a supernova explosion.
Heavy Metals in Stars
Heavy metals in stellar composition
In addition to fusing hydrogen and helium, stars also fuse heavier elements like carbon, oxygen, and iron in their hot, dense cores. Astronomers broadly refer to all elements heavier than helium as “metals” since most elements from lithium and above on the periodic system are metals in their pure form.
So to astronomers, oxygen, gold, sulfur, and all heavy elements count as “metals”, even if some also exhibit non-metallic chemistry. The proportion of these heavy atoms compared to primordial hydrogen and helium is called a star’s metallicity.
Younger stars feature higher metallicities since heavier elements increased as aging stars fused more materials, later dispersing them into space as gas clouds. The changing stellar metal abundances influence luminosity and lifespan over cosmic history.
The origins and evolving impact of heavy metals serve as diagnostics of fusion and enrichment processes central to our universe’s stellar lifetimes.
Fusion of Hydrogen to Heavier Elements
Inside stars, immense heat and pressure fuse hydrogen atoms together. This nuclear fusion process releases energy carried by light outwards. It also creates slightly heavier helium nuclei within the core.
Fusion requires extreme temperatures of millions of degrees to overcome electric repulsion between hydrogen nuclei. Only the dense cores of stars reach these drastic temperatures.
How hydrogen fuses to form heavier elements
Hydrogen atoms fuse into more stable helium by multiple reaction chains. The proton-proton chain fuses hydrogen directly to helium-4 by steps. The CNO cycle uses carbon, oxygen, and nitrogen to enable the fusion process.
These reactions build up helium ash at the core over billions of years. Gradually, heavier elements can form as helium fuses. The process culminates in iron before energy loss halts fusion.
The proton-proton chains and CNO cycles require extremely high temperatures and densities only found in stellar cores, making fusion a gradual process of building up metals. Heavier nuclei like iron mark the point where fusion no longer releases net energy.
Properties of Star Materials
General properties of materials within stars
The extreme heat, pressure, and gravitational forces inside stars give their constituent gasses, plasmas, and elements exotic properties unlike everyday materials. Understanding these properties helps explain stellar structure.
Matter in stars cannot be directly sampled, so models of temperature, density, composition, and more derive estimates. Spectral analysis also constrains stellar innards.
How stellar properties influence internal matter
Extreme stellar core conditions enable exotic states, like metallic hydrogen plasmas, not found naturally on Earth. Complex interactions between pressure, temperature, density, and composition emerge.
These influential plasma properties change as stars evolve, altering fusion pathways that can dramatically impact energy production, instability, and stellar lifetimes.
The extreme densities compact atoms into quantum electron degenerate matter, governing electrical and thermal conductivity while dictating element creation through fusion processes. This leads to emergent chaotic dynamos regulating stellar stability.
Mass and Density of Star Material
A star’s mass and density vary greatly between its blazing core and its cool outer layers. Density peaks at the crushingly compact core, then drops approaching the surface where gasses turn thin and wispy.
More massive stars up to 100+ times the Sun’s mass contain significantly denser cores fueled by more powerful fusion, while low-mass red dwarfs have weaker, less dense centers.
How mass and density impact stellar structure and behavior
High-density cores maintain stability against gravity through electron degeneracy pressure or thermal pressure from fusion, preventing collapse. More density means more collisions and reactions.
Mass and density influence convection currents, opacity, energy transport, magnetic fields, and pulsations tied to observed properties like brightness and color.
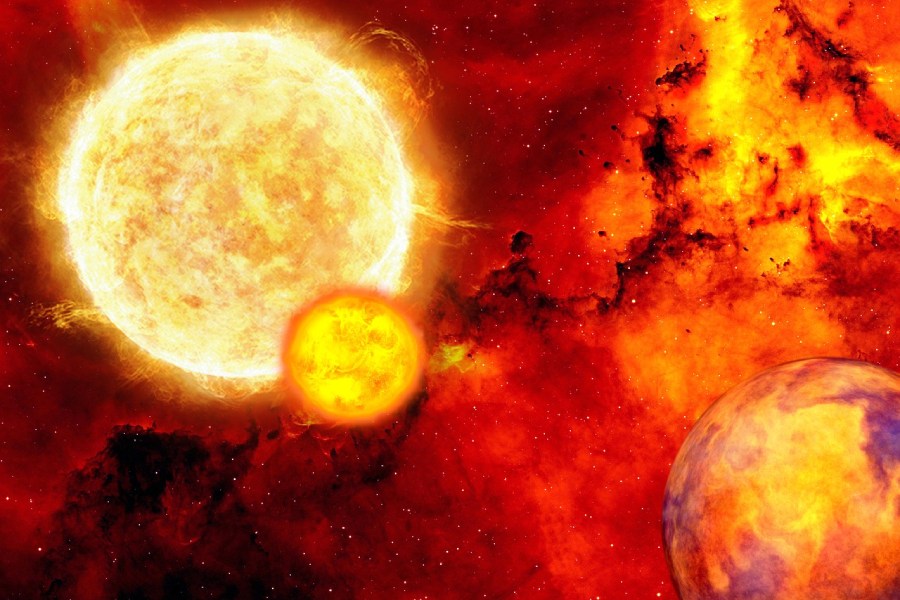
Stellar Nucleosynthesis
Inside stars, hydrogen fuses into helium through nuclear fusion. This process releases energy and forms new atomic nuclei. Fusion requires extremely high densities and temperatures to overcome electric repulsion.
Most fusion occurs in the dense cores of main sequence stars like our Sun. Here, fusion balances gravity to prevent collapse over billions of years.
A detailed discussion of hydrogen fusion in the core
Hydrogen fusion into helium occurs through proton-proton chain reactions and the carbon-nitrogen-oxygen (CNO) cycle. These processes fuse hydrogen nuclei into more stable helium by intermediate steps.
Energy releases heat surrounding gasses, producing pressure and thermal currents. Photons also escape as starlight that we observe here on Earth. Our Sun fuses 600 million tons of hydrogen each second.
After hydrogen fusion declines, aging stars begin fusing helium ashes. In massive stars, additional fusion can produce carbon, oxygen, neon, magnesium, and silicon up to iron before energy losses halt the process.
Supernova explosions of short-lived massive stars disperse freshly fused metals into space. These expelled elements enrich interstellar gas clouds with heavy elements and complex molecules. The enriched gas clouds then condense to form new stars with higher initial metallicity.
The late unstable fusion phases in massive stars create elements heavier than iron. This happens through the absorption of additional neutrons before the star collapses. Finally, the star detonates in a powerful supernova shock wave propagating through surrounding space.
Stellar Structure
A star’s interior structure includes a dense central core where fusion occurs, surrounding radiative and convective zones that transport energy, and thinning outer gas layers.
This differentiation arises because temperature, pressure, and composition vary greatly from the crushingly hot fusion core to the cool diffuse gasses farther out.
Temperature and pressure variations across these layers
At a star’s center, core temperatures can reach 15 million K, and pressures exceed 250 billion times Earth’s atmosphere. But surface temperatures are just thousands of degrees with little pressure.
Shockwaves also propagate outwards from the reactive core through stellar interiors, altering local density structures over time.
The immense temperature gradient from the fusion core to the photosphere drives convection, as photons take eons to diffuse outward through increasingly opaque plasma gradients radiatively. This sets up currents that critically regulate energy flow and stability.
How these layers contribute to the overall structure of a star
The dense core, which is contained by the thick, hot plasma layers, provides a stable energy source and prevents gravitational collapse. Convective and radiative zones move energy up through opacity gradients via particle motions and radiation.
The spreading outer layers act as the photosphere and atmosphere that display characteristic stellar spectra and evolution for classification based on internal structural differences.
Energy Production in Stars
How stars generate energy
Nuclear fusion of hydrogen into helium in stellar cores generates vast quantities of energy according to Einstein’s equation E=mc2. Fusing small amounts of mass releases tremendous energy. Temperature and density conditions in stellar interiors unlock the large energy reserves contained within nuclear bonds between protons and neutrons.
Every fusion reaction in stellar cores decreases the mass of the reacting hydrogen and helium nuclei. This lost matter gets converted into radiant energy that streams outward from the core. Einstein’s equation of mass-energy equivalence (E=mc^2) explains this process, where matter transforms into energy.
Conversion of mass to energy in the core
During fusion, a small percentage of mass gets converted to energy as the strong nuclear force reconfigures nucleons into more stable arrangements. The energy rapidly disperses outward from the core.
For example, our Sun converts 4 million tons of matter into energy every second through fusion processes. This enormous energy powers the Sun and Solar System.
Role of nuclear fusion in releasing energy within stars
Without ongoing fusion in stellar cores, existing energy would be rapidly lost through the stellar surface. This would lead to core collapse under gravity in just millions of years.
Instead, sustained fusion maintains equilibrium inside stars for billions of years by continually replenishing radiant energy supplies that balance the crushing inward forces.
When fusion fuel is exhausted, the core loses thermal pressure support, allowing electron degeneracy or neutron degeneracy pressure as density rises before collapse progresses into later burning phases or the final endpoints like dwarfs or supernovae.
Conclusion
Through this article, we hope you better understand what is a star made of. By exploring star nurseries, nuclear furnaces, and the journey of gasses into starlight, we uncovered the raw materials and physics shaping stars.
We followed fusion chains building heavy metals and energy migrating from churning cores of massive stars and tiny dwarfs.
From primordial hydrogen to brilliant supernovae dispersing newly fused fragments, you see stars transform common atoms into dazzling cosmic jewels lighting up the vast dark space.