Cosmic radiation, a pervasive and mysterious force permeating the universe, holds profound significance in space exploration and the cosmos. As humanity ventures further into space, understanding and mitigating the effects of cosmic radiation becomes increasingly crucial. So, what is cosmic radiation? Is cosmic radiation harmful?
We’ll explore the origins of these mysterious rays emanating from stellar engines, collapsed stars, and colossal explosions scattered throughout the galaxies. Discover the sources of cosmic radiation, the various types of particles it comprises, and the staggering energy they possess.
As we unpack the discovery of galactic cosmic radiation, we will shed new light on what these exotic rays from across the stars truly represent. Let’s start!
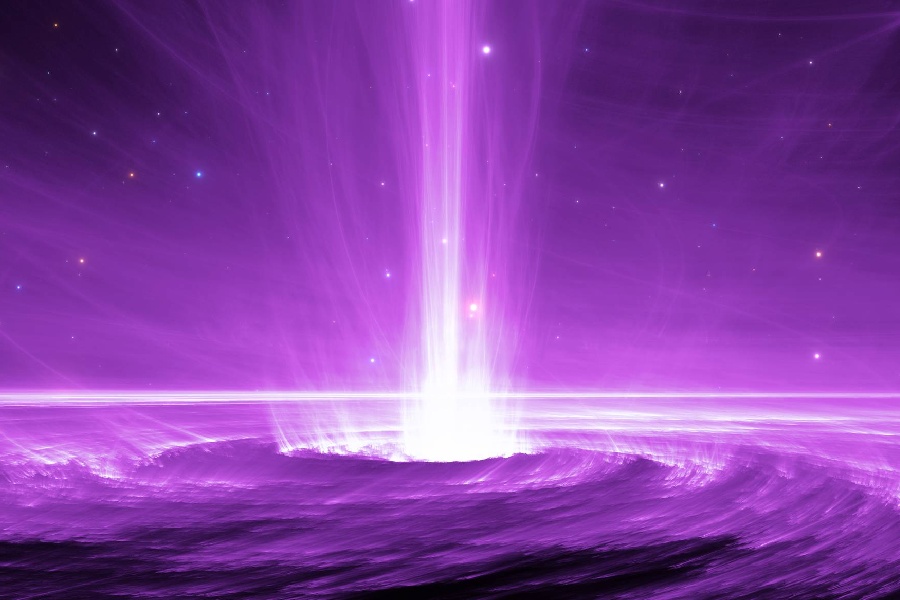
What Is Cosmic Radiation?
Cosmic radiation is composed of energetic particles, x-rays and gamma rays, which travel through space, emanating from various sources, including the Sun and distant stars. Like protons and electrons, these particles can also come from beyond our galaxy. Upon interaction with Earth’s atmosphere, they generate secondary particles and radiation.Â
Fortunately, most cosmic radiation is benign and intercepted by the protective layers of our planet. Yet, astronauts in space or individuals aboard high-altitude flights may encounter heightened exposure levels, potentially leading to health hazards.
Types of Cosmic Radiation
Our cosmos is filled with various types of radiation extending beyond visible light. Scientists study these energetic emissions in space to uncover secrets about the fundamental forces governing the universe. So, here are the types of radiation in space:
Alpha particles
Alpha particles are composed of two protons and two neutrons tightly bound together, resembling ionized helium nuclei. They are emitted during the radioactive decay of heavy elements and can also be produced by collisions of cosmic particles.
While materials can effectively block alpha particles, their energetic radiation can still cause damage to sensitive electronics and human cells through ionization. Monitoring changes in alpha particle flux provides valuable insights into solar cycles and helps scientists understand how cosmic rays travel through magnetic fields surrounding stars.
In space missions, assessing radiation exposure relies on measuring alpha emission rates. These particles are doubly ionized, leaving distinctive spectroscopic signatures. This aids in the identification of their original source isotopes, whether they are naturally occurring or of artificial origin, across the universe.
Solar wind
The Sun emits a constant outward stream of charged particles dubbed the solar wind. This energetic radiation flows through the dimensions of the Solar System, compressing planetary magnetospheres and fueling the shimmering aurora light shows.
Changes in solar wind fluxes link to storm activity and cycles on the Sun, which substantially impact space communications and astronaut safety. By mapping solar wind effects across different planets and moons over time.
The accumulated surface ionization permits estimating the relative ages of surface features. It also provides radiation sheltering to any subsurface life present as robots and humans extend across the Solar System.
Moreover, continuously monitoring solar wind gives in advance alerts. These alerts prompt taking protective measures against intensified gusts. Enhanced solar weather spells may occur. Monitoring also informs models about the dynamics of Mars‘ long-lost thicker atmosphere.
Solar flares
Solar flares, like intense bursts of magnetic energy on the Sun, emit floods of light particles and bands of X-rays. These eruptions influence space weather fluctuations and pose risks to electronics. Satellites and manned spacecraft orbiting Earth face potential failures due to intense proton bombardment and electromagnetic pulses from solar flares.
To mitigate these risks, predicting solar flares and fortifying electronic systems against their effects are crucial. This resilience is vital for humanity’s efforts to explore deeper into space. Additionally, immense solar flares produce energetic photons that can threaten astronaut health unless they are properly shielded or located in protected areas.
As human ambitions turn towards extended stays on Mars and long-range human-crewed expeditions, understanding solar storms becomes essential. These storms profoundly impact planets, making solar physics a critical domain for safe exploration.
Secondary particles
Galactic cosmic ray protons, like powerful gusts of wind, collide with planetary atmospheres and icy moon surfaces. Upon impact, they create cascading showers of secondary subatomic particles in the air and clouds, known as secondary particles.
These secondary particles generate extensive air showers, which consist of branching fragmentation spirals. These showers produce abundant energetic photons, electrons, neutrons, and muons.
Understanding the distribution and fallout of these secondary atmospheric particles is crucial. It provides detailed insights into the original composition and intensity spectrum of the cosmic ray protons that initiated them. This information is vital for developing accurate radiation dosage and shielding models.
Secondary shower sprites, which can travel for miles, pose significant but often underestimated threats. While shielding typically focuses on the heavier primary particles that penetrate less deeply, it’s important also to consider these secondary threats.
Energetic particles
As stars near the end of their lives, powerful shockwaves erupt from exploding supernovae. These shockwaves propel common interstellar particles, such as protons and electrons, to incredibly high speeds, nearing that of light.
These newly energized cosmic rays carry significantly more momentum and harmful radiation compared to the usual thermal energy of particles in space. They are widespread throughout galaxies, influencing the creation of complex molecules essential for life.
These energetic cosmic rays play a role in potentially making planets habitable. When they interact with atmospheres and surfaces, they might contribute to the conditions suitable for life to thrive.
Additionally, scientists theorize that unique cosmic particles resulting from dark matter annihilation could be detected, providing insights into the mysterious nature of dark matter. Discovering the true mediators of dark matter would revolutionize our understanding of the structure and origins of the cosmos.
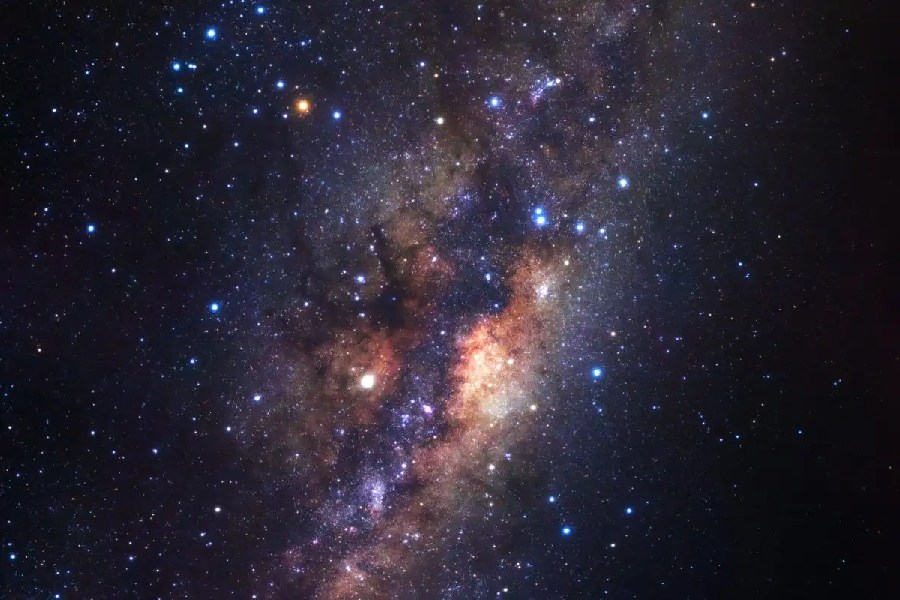
Detection and Measurement
Detection methods
Various instruments are used to detect cosmic radiation types and energies, ranging from ground-based observatories to space. Neutron monitors track secondary air shower particle signatures to trace solar storms and galactic cosmic rays from light years away.
Furthermore, satellite X-ray and gamma-ray detectors create dynamic maps of high-energy celestial sources and track flux changes over time. Large radio telescope arrays document distant pulsars, quasars, and stellar particle accelerators through their emissions.
These diverse cosmic signals contribute to evidence-based models. The models might predict hazards posed by space radiation to electronics and human health.
Assessing exposure
Personal radiation badges are equipped with radiochromic film, enabling them to monitor individual and environmental cosmic ray exposure during space missions beyond Earth’s protective magnetic field. The film changes color over time, reflecting the cumulative ionizing radiation dose received. This radiation dose originates from direct exposure to charged particles and indirect exposure to secondary neutron fluxes.
To ensure the safety of astronauts during extended missions beyond Earth’s natural protection, strict legal limits on radiation exposure are combined with thorough assessments of habitat and vehicle shielding effectiveness. The goal is to minimize the risk of stochastic effects and acute cell damage.
During long tours of duty in space, astronauts rely on robotics to maintain satellite connections and conduct exploration activities. These robots serve a vital role by absorbing radiation that would otherwise damage electronics and sensors, reducing the risk to the biological crew.
Effects of Cosmic Radiation
Now that we’ve understood cosmic radiation and its detection methods, it’s important to consider the risks it presents. Let’s explore how these invisible energies affect both machines and humans.
Increased particle energies
Upon initial contact, a cosmic ray proton collides with atoms and molecules within planetary atmospheres or dense structural materials of crew spacecraft. This collision significantly increases the kinetic energy and momentum of the constituent particles involved.
This instantaneously raises electrons and atomic nucleus fragments to fleeting velocities near light speed. The collision also excites quarks inside, as neutrons and protons are shredded during the process. Such radical interactions trigger an expanding particle shower cascade, including transient pions, muons, and cascading electrons.
The swiftly growing radial particle spike holds the greatest biological hazard. Remarkably, deep atmospheric or spacecraft hull penetration transports chaotic damage far from the original cosmic ray entry channel.
Cellular damage
When cosmic rays focus on small areas within a spacecraft or habitat, they can cause concentrated cascades of fast-moving particles, possibly putting astronauts at risk of DNA damage. This might increase the chances of mutations or even cancer over time.
However, secondary particle radiation from cosmic rays commonly passes through human tissue to other targets. This radiation might damage neurons, accumulating over an astronaut’s career. Since this gradual neurological disruption can’t be shielded or repaired, exposure must be limited through prudent mission planning to ensure safety.
As space travel goals expand, it’s crucial to continually improve assessments of cumulative cosmic radiation impacts on cells and DNA. This will guide ethical considerations and readiness in pushing humanity’s reach farther into space.
Shielding requirements
To protect astronauts from the harmful effects of cosmic rays, thick shielding is essential around crew areas. Yet, the secondary particles produced by these rays can penetrate even through shielding layers.
Computer models play a crucial role in designing effective partitions between workspaces and dense water walls, which mimic the composition of asteroids. These models help find the best configurations to minimize crews’ exposure to both primary and secondary cosmic radiation.
As missions venture further, such as to Mars, cosmic ray exposure increases, potentially reaching the maximum total dose limits within two to three years. This emphasizes the significant challenge of energetic solar and galactic particles during space missions.
Conclusion
What is cosmic radiation? To sum up, it is vital to space exploration, shedding light on the universe’s mysteries. So, we explored its origins, types, and effects, revealing its challenges to astronauts and spacecraft.
As humanity ventures deeper into space, understanding and mitigating cosmic radiation’s impact become paramount. Through ongoing research and innovation, we aim to improve shielding techniques and detection methods for safer space exploration.
The exploration of cosmic radiation shows the interconnectedness of celestial phenomena, guiding us toward safer space endeavors. As we continue this journey, let’s harness our understanding to propel us toward a brighter future among the stars, where exploration knows fewer boundaries.