Gazing up at the cosmos, the planets of our Solar System appear like spheres of rock and gas spinning through space. The spherical nature of planets has long intrigued scientists and stargazers alike. This fascination stems from the fundamental forces that govern celestial bodies. So, why planets are round? Do all planets have gravity?
In this article, we’ll discover why planets are round and unpack the science behind the spherical shapes defining the worlds in Earth’s orbital neighborhood and beyond. Understanding the planetary grinding and molding processes that tend toward spheres will show why a reliable geometric regularity emerges from accretion disk chaos.
We’ll also learn about the physics underlying orb-like worlds and why exceptions like Halley’s Comet arrive lumpy and misshapen by comparison. Let’s start!
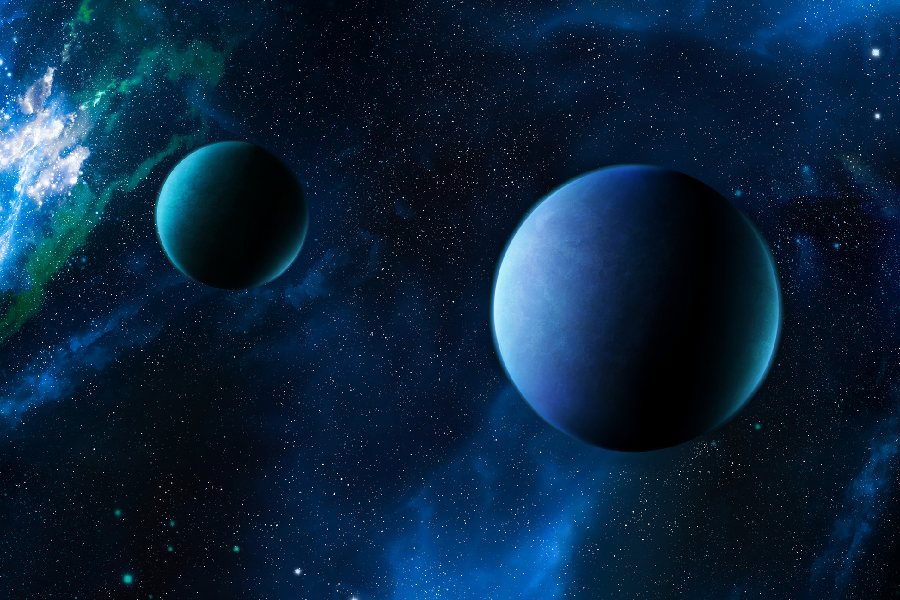
Why Planets Are Round in Shape?
Planets are round primarily because of the force of gravity. During their formation from a protoplanetary disk, material is drawn together by gravity, creating a roughly spherical shape.
The equilibrium between gravitational forces and the planet’s rotation results in a stable, spherical structure. While factors like composition and rotation speed can introduce some variations, gravity tends to shape planets into round forms.
This roundness is crucial for stability and mass distribution, making it the natural and efficient form for planets. The force of gravity shapes these celestial bodies into spheres, a characteristic observed throughout the universe.
The spherical nature of planets
Planets form into spheres as they come together from surrounding gas and dust after their stars ignite. But are planets perfect spheres? Larger planets have enough gravity to smooth out any initial jagged shapes from rocks or ice.
Spheres minimize the gravitational potential energy for the quantity of mass making up each planet. This efficient shape naturally happens as particles merge under gravity and geology changes surfaces over time.
Spheres enable hydrostatic equilibrium, where incredibly strong compressive forces balance within flowing hot interiors for billions of years. So, spheres emerge as the default contour for mature planets and moons, from small icy moons to giant gaseous worlds.
Structures often deviate from roundness only when material strength succumbs to gravity, as seen in Saturn’s rings. The spherical shape, on the other hand, ensures internal stability and facilitates smooth spinning.
Gravitational pull
A planet’s gravity pulls material in evenly from all sides toward its center. This squeezes any initial irregular chunks into a more compact shape over time. Gravity works to gather a planet’s mass into the smallest-volume form possible, given the amount of matter involved.
It pulls harder on areas sticking out farther from the center. Hence, protruding regions get drawn back more than surrounding terrain. Impacts and geology gradually smooth things out toward a sphere.
In a sphere, gravitational forces fully balance across all directions. But do all planets have gravity acting to round them this way? This constant compressive force comes from any planet’s sizable mass, crushing worlds toward spherical symmetry.
Massive bodies, shaped by their gravitational pull, gradually settle into stable spheres over time. This persistent force across the planet continues to mold celestial bodies into reliable globes.
Initial conditions
Raw materials and the surrounding environment influence the formation of planets, ultimately shaping them into spheres. A spinning accretion disk from a parent star ensures early rotation during the coalescing process. Lighter gases contribute to the early rounding, while solid impacts can leave lasting surface marks.
As a star system forms, the swirling dust and gas impact the motions and densities of particles, influencing the development of planets. Temperature variations from the central star affect the minerals that form. Explosive impacts during this process create additional irregularities.
Gravity plays a role over time, pulling mature planets into spherical shapes. However, the initial chance events result in small variations that persist today. Internal density patterns reveal ancient crashes; slight tilts during planetary spin expose their disorganized beginnings.
Hydrostatic equilibrium
Hydrostatic equilibrium explains how spheres maintain stability under high interior pressure. It involves balancing the inward force of gravity against outward pressure gradients.
Equilibrium happens when gravity tries compressing a planet’s fluid insides. However, increasing pressure resists further compression, creating an outward gradient. This self-perpetuating physics emerges for large accumulating masses. It allows spheres to settle naturally once compression balances across layered interiors.
So, over time, hydrostatic forces fine-tune roundness by smoothing deviations that would stretch equilibrium. Planets gravitate toward durable spheres thanks to this give-and-take that sustains harmony between intense, complex forces.
Planet bulge
As planets spin, centrifugal forces cause a slight outward bulge around the equator. Speeds increase away from the central axis of rotation, causing more mass to be pushed outward.
This effect subtly stretches the sphere shape. Jupiter and Saturn show small distinct equatorial bulges due to their rapid rotation nearing breakup speed. Yet, the spin-related distortion remains stable without hampering overall roundness.
A mature planet’s tiny bulge aligns with its spin plane for billions of years. Seasonal heating variations can also slightly stretch spheres from perfect uniformity based on changing solar angles during orbital cycles. Nonetheless, global roundness holds strong with only the subtlest of deformations arising.
Perfect Sphere and Shape
Planets gradually develop a spherical shape, but achieving perfect geometric roundness proves challenging due to internal and external factors. Only when there is uniform density can a truly “perfect” sphere take shape.
In reality, worlds are filled with intricate structures and processes, preventing absolute smoothness. Minor yet meaningful deviations arise, including collisions, ring systems, and other influences. Despite these variations, maintaining overall consistency is crucial for maintaining equilibrium.
Galilean moons
In 1610, Galileo observed Jupiter’s four Galilean moons using early telescopes. Confirming their spherical shapes, these moons vary in size and composition as they orbit the giant planet.
Io, one of Jupiter’s moons, exhibits extreme volcanism that frequently reshapes its surface. Meanwhile, Europa, Ganymede, and Callisto have icy crusts preserving ancient terrains with cracks and crater marks.
Studying this mini-planetary system helps us understand how interior melting and external collisions influence the formation of spherical worlds. The layered interior structures of these dwarf planets offer valuable insights. The resonant orbits of these dwarf planets also provide insights into the processes that smooth celestial bodies into spherical shapes.
Conclusion
Fundamental physics underlies why planets are round and celestial bodies like planets tend to take on spherical shapes. Factors from accretion disk momentum to gravitational pulling and geological smoothing work over billions of years to negate irregularities and approach smooth orbital globes.
Achieving hydrostatic equilibrium enables this inherent spherical nature as gravitational compression balances internal pressure resistance. Small deviations may emerge, but gravity and pressure gradients perpetually guide formation toward the optimal contour equilibrium of spheres.
We hope tracing the significance of hydrostatic forces to gravitational pulls has illuminated the planetary rounding processes. These processes transform lumpy proto-worlds into reliable orbs across our Solar System and beyond.